Advances in solid adsorbent materials for direct air capture of CO2
DOI:
https://doi.org/10.18686/cest.v1i2.95Keywords:
direct air capture of CO2; adsorbent; solution absorption; solid adsorption; economic efficiencyAbstract
Direct air capture (DAC) of CO2 is a carbon-negative technology that is not limited by time and geography and can contribute to the realization of the “dual-carbon” goal. In this paper, the current development of direct air capture of CO2 is reviewed, focusing on four mainstream solid adsorption DAC technologies, namely, metal-organic frameworks (MOFs), solid amine materials, alkali-metal-based adsorbents, and moisture-altering materials, and their advantages and disadvantages in terms of energy consumption, cyclic stability, and adsorption capacity are analyzed. In addition, the engineering applications of solid adsorbent materials are analyzed and the potential of these technologies in practical applications is demonstrated. Finally, the challenges faced by existing DAC adsorbent materials are summarized and the future development direction is put forward.
References
1. Ekholm N. On the variations of the climate of the geological and historical past and their causes. Quarterly Journal of the Royal Meteorological Society 1901; 27(117): 1–62. doi: 10.1002/qj.49702711702
2. Mackay A. Climate change 2007: Impacts, adaptation and vulnerability. contribution of working group ii to the fourth assessment report of the intergovernmental panel on climate change. Journal of Environmental Quality 2008; 37(6): 2407. doi: 10.2134/jeq2008.0015br
3. Field CB, Barros VR, Dokken DJ, et al. Climate Change 2014 Impacts, Adaptation, and Vulnerability. Part A: Global and Sectoral Aspects. Cambridge University Press; 2014.
4. Kiehl JT, Trenberth KE. Earth’s annual global mean energy budget. Bulletin of the American Meteorological Society 1997; 78(2): 197–208. doi: 10.1175/1520-0477(1997)078<0197:EAGMEB>2.0.CO;2
5. China Meteorological Administration. The World Meteorological Organization releases “State of the Global Climate 2021”—Four key indicators: Greenhouse gas concentration, sea level rise, ocean heat, and ocean acidification hit records (Chinese). Available online: https://www.cma.gov.cn/2011xwzx/2011xqxxw/2011xqxyw/202205/t20220523_4846229.html (accessed on 27 November 2023).
6. Minx JC, Lamb WF, Callaghan MW, et al. Negative emissions— Part 1: Research landscape and synthesis. Environmental Research Letters 2018; 13(6): 063001. doi: 10.1088/1748-9326/aabf9b
7. Xinhua News Agency. “Opinions of the Central Committee of the Communist Party of China and the State Council on Completely, Accurately and Comprehensively Implementing the New Development Concept and Doing a Good Job in Carbon Peaking and Carbon Neutralization” was released (Chinese). Resources and Habitant Environment 2021; 2021(11): 6.
8. Huang J. Coupled Research on CO2 Air Capture and Conversion Utilization (Chinese) [Master’s thesis]. Zhejiang University; 2016.
9. Lackner K, Ziock HJ, Grimes P. Carbon dioxide extraction from air: Is it an option? In: Proceedings of the 24th Annual Technical Conference on Coal Utilization and Fuel Systems; 8–11 March 1999; Clearwater, FL, US. pp. 885–896.
10. Keith DW, Ha-Duong M, Stolaroff JK. Climate strategy with CO2 capture from the air. Climatic Change 2006; 74(1–3): 17–45. doi: 10.1007/s10584-005-9026-x
11. Sutherland BR. Pricing CO2 direct air capture. Joule 2019; 3(7): 1571–1573. doi: 10.1016/j.joule.2019.06.025
12. Lackner KS. Capture of carbon dioxide from ambient air. The European Physical Journal Special Topics 2009; 176: 93–106. doi: 10.1140/epjst/e2009-01150-3
13. Sanz-Pérez ES, Murdock CR, Didas SA, et al. Direct capture of CO2 from ambient air. Chemical Reviews 2016; 116(19): 11840–11876. doi: 10.1021/acs.chemrev.6b00173
14. Lackner KS, Brennan S, Matter JM, et al. The urgency of the development of CO2 capture from ambient air. Proceedings of the National Academy of Sciences 2012; 109(33): 13156–13162. doi: 10.1073/pnas.1108765109
15. Eden J. IEA: Carbon capture crucial to limit global warming. Oil Daily 2020; 2020: 5–6.
16. Zeman F. Reducing the cost of Ca-based direct air capture of CO2. Environmental Science & Technology 2014; 48(19): 11730–11735. doi: 10.1021/es502887y
17. Zeman F. Experimental results for capturing CO2 from the atmosphere. AIChE Journal 2008; 54(5): 1396–1399. doi: 10.1002/aic.11452
18. Baciocchi R, Storti G, Mazzotti M. Process design and energy requirements for the capture of carbon dioxide from air. Chemical Engineering and Processing: Process Intensification 2006; 45(12): 1047–1058. doi: 10.1016/j.cep.2006.03.015
19. Zeman F. Energy and material balance of CO2 capture from ambient air. Environmental Science & Technology 2007; 41(21): 7558–7563. doi: 10.1021/es070874m
20. Stolaroff JK, Keith DW, Lowry GV. Carbon dioxide capture from atmospheric air using sodium hydroxide spray. Environmental Science & Technology 2008; 42(8): 2728–2735. doi: 10.1021/es702607w
21. Mahmoudkhani M, Heidel KR, Ferreira JC, et al. Low energy packed tower and caustic recovery for direct capture of CO2 from air. Energy Procedia 2009; 1(1): 1535–1542. doi: 10.1016/j.egypro.2009.01.201
22. Mahmoudkhani M, Keith DW. Low-energy sodium hydroxide recovery for CO2 capture from atmospheric air—Thermodynamic analysis. International Journal of Greenhouse Gas Control 2009; 3(4): 376–384. doi: 10.1016/j.ijggc.2009.02.003
23. Sabatino F, Grimm A, Gallucci F, et al. A comparative energy and costs assessment and optimization for direct air capture technologies. Joule 2021; 5(8): 2047–2076. doi: 10.1016/j.joule.2021.05.023
24. Keith DW, Holmes G, Angelo DS, Heidel K. A process for capturing CO2 from the atmosphere. Joule 2018; 2(8): 1573–1594. doi: 10.1016/j.joule.2018.05.006
25. Holmes G, Nold K, Walsh T, et al. Outdoor prototype results for direct atmospheric capture of carbon dioxide. Energy Procedia 2013; 37: 6079–6095. doi: 10.1016/j.egypro.2013.06.537
26. Brethomé FM, Williams NJ, Seipp CA, et al. Direct air capture of CO2 via aqueous-phase absorption and crystalline-phase release using concentrated solar power. Nature Energy 2018; 3(7): 553–559. doi: 10.1038/s41560-018-0150-z
27. Custelcean R, Williams NJ, Garrabrant KA, et al. Direct air capture of CO2 with aqueous amino acids and solid bis-iminoguanidines (BIGs). Industrial & Engineering Chemistry Research 2019; 58(51): 23338–23346. doi: 10.1021/acs.iecr.9b04800
28. Wang D, Zhang J, Yang B, Wu Z. Research progress of typical process analysis and techno-economic research on direct air capture of carbon dioxide. Coal Science and Technology 2023; 51(S1): 215–221. doi: 10.13199/j.cnki.cst.2022-0236
29. Wang Q, Luo J, Zhong Z, Borgna A. CO2 capture by solid adsorbents and their applications: Current status and new trends. Energy & Environmental Science 2011; 4(1): 42–55. doi: 10.1039/C0EE00064G
30. Wang H, Qu ZG, Bai JQ, Qiu YS. Combined grand canonical Monte Carlo and finite volume method simulation method for investigation of direct air capture of low concentration CO2 by 5A zeolite adsorbent bed. International Journal of Heat and Mass Transfer 2018; 126: 1219–1235. doi: 10.1016/j.ijheatmasstransfer.2018.06.052
31. Stuckert AN, Yang RT. CO2 capture from the atmosphere and simultaneous concentration using zeolites and amine-grafted SBA-15. Environmental Science & Technology 2011; 45(23): 10257–10264. doi: 10.1021/es202647a
32. Wilson SMW, Tezel FH. Direct dry air capture of CO2 using VTSA with faujasite zeolites. Industrial & Engineering Chemistry Research 2020; 59(18): 8783–8794. doi: 10.1021/acs.iecr.9b04803
33. D’Alessandro D, Smit B, Long J. Carbon dioxide capture: Prospects for new materials. Angewandte Chemie International Edition 2010; 49(35): 6058–6082. doi: 10.1002/anie.201000431
34. Rangnekar N, Mittal N, Elyassi B, et al. Zeolite membranes—A review and comparison with MOFs. Chemical Society Reviews 2015; 44(20): 7128–7154. doi: 10.1039/C5CS00292C
35. Yu J, Xie LH, Li JR, et al. CO2 capture and separations using MOFs: Computational and experimental studies. Chemical Reviews 2017; 117(14): 9674–9754. doi: 10.1021/acs.chemrev.6b00626
36. Saha D, Bao ZB, Jia F, Deng S. Adsorption of CO2, CH4, N2O, and N2 on MOF-5, MOF-177, and zeolite 5A. Environmental Science & Technology 2010; 44(5): 1820–1826. doi: 10.1021/es9032309
37. Oschatz M, Antonietti M. A search for selectivity to enable CO2 capture with porous adsorbents. Energy & Environmental Science 2018; 11(1): 57–70. doi: 10.1039/C7EE02110K
38. Nugent P, Belmabkhout Y, Burd SD, et al. Porous materials with optimal adsorption thermodynamics and kinetics for CO2 separation. Nature 2013; 495(7439): 80–84. doi: 10.1038/nature11893
39. Shekhah O, Belmabkhout Y, Adil K, et al. A facile solvent-free synthesis route for the assembly of a highly CO2 selective and H2S tolerant NiSIFSIX metal-organic framework. Chemical Communications 2015; 51(71): 13595–13598. doi: 10.1039/C5CC04487A
40. Shekhah O, Belmabkhout Y, Chen Z, et al. Made-to-order metal-organic frameworks for trace carbon dioxide removal and air capture. Nature Communications 2014; 5: 4228. doi: 10.1038/ncomms5228
41. Ding M, Flaig RW, Jiang HL, Yaghi OM. Carbon capture and conversion using metal-organic frameworks and MOF-based materials. Chemical Society Reviews 2019; 48(10): 2783–2828. doi: 10.1039/C8CS00829A
42. Kumar A, Madden DG, Lusi M, et al. Direct air capture of CO2 by physisorbent materials. Angewandte Chemie International Edition 2015; 54(48): 14372–14377. doi: 10.1002/anie.201506952
43. Madden DG, Scott HS, Kumar A, et al. Flue-gas and direct-air capture of CO2 by porous metal-organic materials. Philosophical Transactions of the Royal Society A—Mathematical Physical And Engineering Sciences 2017; 375(2084): 20160025. doi: 10.1098/rsta.2016.0025
44. Prestipino C, Regli L, Vitillo JG, et al. Local structure of framework Cu(II) in HKUST-1 metallorganic framework: Spectroscopic characterization upon activation and interaction with adsorbates. Chemistry of Materials 2006; 18(5): 1337–1346. doi: 10.1021/cm052191g
45. Liu J, Wei Y, Zhao Y. Trace carbon dioxide capture by metal-organic frameworks. ACS Sustainable Chemistry & Engineering 2019; 7(1): 82–93. doi: 10.1021/acssuschemeng.8b05590
46. McDonald TM, Lee WR, Mason JA, et al. Capture of carbon dioxide from air and flue gas in the alkylamine-appended metal-organic framework mmen-Mg-2(dobpdc). Journal of The American Chemical Society 2012; 134(16): 7056–7065. doi: 10.1021/ja300034j
47. Zhang Z, Ding Q, Cui J, et al. High and selective capture of low-concentration CO2 with an anion-functionalized ultramicroporous metal-organic framework. Science China-Materials 2021; 64(3): 691–697. doi: 10.1007/s40843-020-1471-0
48. Lee WR, Hwang SY, Ryu DW, et al. Diamine-functionalized metal-organic framework: Exceptionally high CO2 capacities from ambient air and flue gas, ultrafast CO2 uptake rate, and adsorption mechanism. Energy & Environmental Science 2014; 7(2): 744–751. doi: 10.1039/C3EE42328J
49. Bhatt PM, Belmabkhout Y, Cadiau A, et al. A fine-tuned fluorinated MOF addresses the needs for trace CO2 removal and air capture using physisorption. Journal of The American Chemical Society 2016; 138(29): 9301–9307. doi: 10.1021/jacs.6b05345
50. Piscopo CG, Loebbecke S. Strategies to enhance carbon dioxide capture in metal-organic frameworks. ChemPlusChem 2020; 85(3): 538–547. doi: 10.1002/cplu.202000072
51. Shi X, Xiao H, Azarabadi H, et al. Sorbents for the direct capture of CO2 from ambient air. Angewandte Chemie International Edition 2020; 59(18): 6984–7006. doi: 10.1002/anie.201906756
52. Sethia G, Sayari A. Comprehensive study of ultra-microporous nitrogen-doped activated carbon for CO2 capture. Carbon 2015; 93: 68–80. doi: 10.1016/j.carbon.2015.05.017
53. Coromina HM, Walsh DA, Mokaya R. Biomass-derived activated carbon with simultaneously enhanced CO2 uptake for both pre and post combustion capture applications. Journal of Materials Chemistry A 2016; 4(1): 280–289. doi: 10.1039/C5TA09202G
54. Sun Q, Li Z, Searles DJ, et al. Charge-controlled switchable CO2 capture on boron nitride nanomaterials. Journal of The American Chemical Society 2013; 135(22): 8246–8253. doi: 10.1021/ja400243r
55. Lu C, Bai H, Wu B, et al. Comparative study of CO2 capture by carbon nanotubes, activated carbons, and zeolites. Energy & Fuels 2008; 22(5): 3050–3056. doi: 10.1021/ef8000086
56. Danckwerts PV. The reaction of CO2 with ethanolamines. Chemical Engineering Science 1979; 34(4): 443–446. doi: 10.1016/0009-2509(79)85087-3
57. Didas SA, Choi S, Chaikittisilp W, Jones CW. Amine-oxide hybrid materials for CO2 capture from ambient air. Accounts of Chemical Research 2015; 48(10): 2680–2687. doi: 10.1021/acs.accounts.5b00284
58. Bollini P, Didas SA, Jones CW. Amine-oxide hybrid materials for acid gas separations. Journal of Materials Chemistry 2011; 21(39): 15100–15120. doi: 10.1039/C1JM12522B
59. Guo M, Wu H, Lv L, et al. A highly efficient and stable composite of polyacrylate and metal-organic framework prepared by interface engineering for direct air capture. ACS Applied Materials & Interfaces 2021; 13(18): 21775–21785. doi: 10.1021/acsami.1c03661
60. Xu X, Song C, Andresen JM, et al. Novel polyethylenimine-modified mesoporous molecular sieve of MCM-41 type as high-capacity adsorbent for CO2 capture. Energy & Fuels 2002; 16(6): 1463–1469. doi: 10.1021/ef020058u
61. Zhao B, Wang J, Wng M, et al. Study on the adsorption performance of resin-based solid amine adsorbent for low concentration CO2 at room temperature (Chinese). Acta Scientiae Circumstantiae 2014; 34(11): 2892–2898.
62. Chaikittisilp W, Kim HJ, Jones CW. Mesoporous alumina-supported amines as potential steam-stable adsorbents for capturing CO2 from simulated flue gas and ambient air. Energy & Fuels 2011; 25(11): 5528–5537. doi: 10.1021/ef201224v
63. Holewinski A, Sakwa-Novak MA, Carrillo JMY, et al. Aminopolymer mobility and support interactions in silica-PEI composites for CO2 capture applications: A quasielastic neutron scattering study. The Journal of Physical Chemistry B 2017; 121(27): 6721–6731. doi: 10.1021/acs.jpcb.7b04106
64. Belmabkhout Y, Serna-Guerrero R, Sayari A. Adsorption of CO2-containing gas mixtures over amine-bearing pore-expanded MCM-41 silica: Application for CO2 separation. Adsorption 2011; 17(2): 395–401. doi: 10.1007/s10450-011-9348-0
65. Brilman DWF, Veneman R. Capturing atmospheric CO2 using supported amine sorbents. Energy Procedia 2013; 37: 6070–6078. doi: 10.1016/j.egypro.2013.06.536
66. Drese JH, Choi S, Lively RP, et al. Synthesis-structure-property relationships for hyperbranched aminosilica CO2 adsorbents. Advanced Functional Materials 2009; 19(23): 3821–3832. doi: 10.1002/adfm.200901461
67. Choi S, Drese JH, Eisenberger PM, Jones CW. Application of amine-tethered solid sorbents for direct CO2 capture from the ambient air. Environmental Science & Technology 2011; 45(6): 2420–2427. doi: 10.1021/es102797w
68. Goeppert A, Czaun M, May RB, et al. Carbon dioxide capture from the air using a polyamine based regenerable solid adsorbent. Journal of The American Chemical Society 2011; 133(50): 20164–20167. doi: 10.1021/ja2100005
69. Geoeppert A, Czaun M, May RB, et al. Carbon dioxide capture from the air using a polyamine based regenerable solid adsorbent. Journal of the American Chemical Society 2011; 133(50): 20164–20167. doi: 10.1021/ja2100005
70. Zeman FS. Air Etraction: The Feasibility of Absorbing Carbon Dioxide Directly from the Atmosphere [PhD thesis]. Columbia University; 2006.
71. Stolaroff JK, Lowry GV, Keith DW. Using CaO- and MgO-rich industrial waste streams for carbon sequestration. Energy Conversion and Management 2005; 46(5): 687–699. doi: 10.1016/j.enconman.2004.05.009
72. Przepiórski J, Czyżewski A, Pietrzak R, Morawski AW. MgO/CaO-loaded activated carbon for carbon dioxide capture: Practical aspects of use. Industrial & Engineering Chemistry Research 2013; 52(20): 6669–6677. doi: 10.1021/ie302848r
73. Hu J, Hongmanorom P, Chirawatkul P, Kawi S. Efficient integration of CO2 capture and conversion over a Ni supported CeO2-modified CaO microsphere at moderate temperature. Chemical Engineering Journal 2021; 426: 130864. doi: 10.1016/j.cej.2021.130864
74. Nikulshina V, Gálvez ME, Steinfeld A. Kinetic analysis of the carbonation reactions for the capture of CO2 from air via the Ca(OH)2-CaCO3-CaO solar thermochemical cycle. Chemical Engineering Journal 2007; 129(1–3): 75–83. doi: 10.1016/j.cej.2006.11.003
75. Nikulshina V, Ayesa N, Galvez ME, Steinfeld A. Feasibility of Na-based thermochemical cycles for the capture of CO2 from air—Thermodynamic and thermogravimetric analyses. Chemical Engineering Journal 2008; 140(1–3): 62–70. doi: 10.1016/j.cej.2007.09.007
76. Nikulshina V, Gebald C, Steinfeld A. CO2 capture from atmospheric air via consecutive CaO-carbonation and CaCO3-calcination cycles in a fluidized-bed solar reactor. Chemical Engineering Journal 2009; 146(2): 244–248. doi: 10.1016/j.cej.2008.06.005
77. Nikulshina V, Steinfeld A. CO2 capture from air via CaO-carbonation using a solar-driven fluidized bed reactor—Effect of temperature and water vapor concentration. Chemical Engineering Journal 2009; 155(3): 867–873. doi: 10.1016/j.cej.2009.10.004
78. Shigemoto N, Yanagihara T, Sugiyama S, Hayashi H. Bench-scale CO2 recovery from moist flue gases by various alkali carbonates supported on activated carbon. Journal of Chemical Engineering Of Japan 2005; 38(9): 711–717. doi: 10.1252/jcej.38.711
79. Lee SC, Choi BY, Lee SJ, et al. CO2 absorption and regeneration using Na and K based sorbents. Studies in Surface Science and Catalysis 2004; 153: 527–530. doi: 10.1016/S0167-2991(04)80307-0
80. Lee SC, Chae HJ, Kwon YM, et al. Characteristics of new potassium-based sorbents prepared with nano-titanium oxide for carbon dioxide capture. Journal of Nanoelectronics and Optoelectronics 2010; 5(2): 212–217. doi: 10.1166/jno.2010.1096
81. Wang Y, Jia L, Mei X, et al. CO2 adsorption mechanism and failure characteristics of supported potassium-based adsorbent (Chinese). Environmental Science & Technology 2019; 42(11): 98–104.
82. Lee SC, Kwon YM, Park YH, et al. Structure effects of potassium-based TiO2 sorbents on the CO2 capture capacity. Topics in Catalysis 2010; 53(7–10): 641–647. doi: 10.1007/s11244-010-9499-3
83. Zhao C, Guo Y, Li C, Lu S. Removal of low concentration CO2 at ambient temperature using several potassium-based sorbents. Applied Energy 2014; 124: 241–247. doi: 10.1016/j.apenergy.2014.02.054
84. Zhao C, Guo Y, Li C, Lu S. Carbonation behavior of K2CO3/AC in low reaction temperature and CO2 concentration. Chemical Engineering Journal 2014; 254: 524–530. doi: 10.1016/j.cej.2014.05.062
85. Zhao CW, Chen X, Zhao C. Carbonation reaction characteristics of potassium-based CO2 absorbent (Chinese). CIESC Journal 2008; 9: 2328–2333.
86. Dong W. Research on Decarbonization/Regeneration Characteristics and Modification Mechanism of Sodium-Based Solid CO2 Absorbent (Chinese) [PhD thesis]. Southeast University; 2015.
87. Masoud N, Clement V, van Haasterecht T, et al. Shedding light on solid sorbents: Evaluation of supported potassium carbonate particle size and its effect on CO2 capture from air. Industrial & Engineering Chemistry Research 2022; 61(38): 14211–14221. doi: 10.1021/acs.iecr.2c01508
88. Derevschikov VS, Veselovskaya JV, Kardash TY, et al. Direct CO2 capture from ambient air using K2CO3/Y2O3 composite sorbent. Fuel 2014; 127: 212–218. doi: 10.1016/j.fuel.2013.09.060
89. Veselovskaya JV, Derevschikov VS, Kardash TY, et al. Direct CO2 capture from ambient air using K2CO3/Al2O3 composite sorbent. International Journal of Greenhouse Gas Control 2013; 17: 332–340. doi: 10.1016/j.ijggc.2013.05.006
90. Veselovskaya JV, Derevschikov VS, Shalygin AS, Yatsenko DA. K2CO3-containing composite sorbents based on a ZrO2 aerogel for reversible CO2 capture from ambient air. Microporous and Mesoporous Materials 2021; 310: 11064. doi: 10.1016/j.micromeso.2020.110624
91. Bali S, Sakwa-Novak MA, Jones CW. Potassium incorporated alumina based CO2 capture sorbents: Comparison with supported amine sorbents under ultra-dilute capture conditions. Colloids and Surfaces A: Physicochemical and Engineering Aspects 2015; 486: 78–85. doi: 10.1016/j.colsurfa.2015.09.020
92. Jeong-Potter C, Abdallah M, Sanderson C, et al. Dual function materials (Ru+Na2O/Al2O3) for direct air capture of CO2 and in situ catalytic methanation: The impact of realistic ambient conditions. Applied Catalysis B: Environmental 2022; 307: 120990. doi: 10.1016/j.apcatb.2021.120990
93. Wang T, Lackner S, Wright A. Moisture swing sorbent for carbon dioxide capture from ambient air. Environmental Science & Technology 2011; 45(15): 6670–6675. doi: 10.1021/es201180v
94. Wang T, Wang X, Hou C, Liu J. Quaternary functionalized mesoporous adsorbents for ultra-high kinetics of CO2 capture from air. Scientific Reports 2020; 10(1): 21429. doi: 10.1038/s41598-020-77477-1
95. Wang T, Ge K, Chen K, et al. Theoretical studies on CO2 capture behavior of quaternary ammonium-based polymeric ionic liquids. Physical Chemistry Chemical Physics 2016; 18(18): 13084–13091. doi: 10.1039/C5CP07229H
96. Wang T, Hou C, Ge K, et al. Spontaneous cooling absorption of CO2 by a polymeric ionic liquid for direct air capture. Journal of Physical Chemistry Letters 2017; 8(17): 3986–3990. doi: 10.1021/acs.jpclett.7b01726
97. Xu S, Hou C,Wang T. Research on CO2 adsorption heat transfer of quaternary ammonium type humidified regenerated materials (Chinese). Energy Engineering 2021; 1: 54–62, 88. doi: 10.16189/j.cnki.nygc.2021.01.009
98. He H, Li W, Zhong M, et al. Reversible CO2 capture with porous polymers using the humidity swing. Energy & Environmental Science 2013; 6(2): 488–493. doi: 10.1039/C2EE24139K
99. Armstrong M, Shi X, Shan B, et al. Rapid CO2 capture from ambient air by sorbent-containing porous electrospun fibers made with the solvothermal polymer additive removal technique. AIChE Journal 2019; 65(1): 214–220. doi: 10.1002/aic.16418
100. Song J, Liu J, Zhao W, et al. Quaternized chitosan/PVA aerogels for reversible CO2 capture from ambient air. Industrial & Engineering Chemistry Research 2018; 57(14): 4941–4948. doi: 10.1021/acs.iecr.8b00064
101. Sun X, Zhu L, Wang P, et al. CO2 removal from natural gas by moisture swing adsorption. Chemical Engineering Research and Design 2021; 176: 162–168. doi: 10.1016/j.cherd.2021.09.033
102. Shayegh S, Bosetti V, Tavoni M. Future prospects of direct air capture technologies: Insights from an expert elicitation survey. Frontiers in Climate 2021; 3: 630893. doi: 10.3389/fclim.2021.630893
103. Xing W, Xu R, Gao H, Duan L. Analysis on the development of technology for direct CO2 capture in the air from a patent perspective (Chinese). Clean Coal Technology 2023; 29(4): 86–97.
104. Wang J, He S, Yan J, et al. Research progress and challenges on life cycle assessment of direct air carbon capture technology (Chinese). Journal of China Coal Society 2023; 48(7): 2748–2759.
105. Bao H, Lin Z, Mao H, et al. Research on configuration optimization of direct air carbon capture and utilization system driven by renewable energy (Chinese). Power System Engineering 2023; 39(3): 1–6.
106. Gutknecht V, Snæbjörnsdóttir SÓ, Sigfússon B, et al. Creating a carbon dioxide removal solution by combining rapid mineralization of CO2 with direct air capture. Energy Procedia 2018; 146: 129–134. doi: 10.1016/j.egypro.2018.07.017
107. Energy Daily. World’s largest device to capture carbon dioxide from air launches in Iceland (Chinese). Sino-Global Energy 2021; 26(12): 88.
108. Sillman J, Nygren L, Kahiluoto H, et al. Bacterial protein for food and feed generated via renewable energy and direct air capture of CO2: Can it reduce land and water use? Global Food Security 2019; 22: 25–32. doi: 10.1016/j.gfs.2019.09.007
109. Sadiq MM, Batten MP, Mulet X, et al. A pilot-scale demonstration of mobile direct air capture using metal-organic frameworks. Advanced Sustainable Systems 2020; 4(12): 2000101. doi: 10.1002/adsu.202000101
110. Ranjan M, Herzog HJ. Feasibility of air capture. Energy Procedia 2011; 4: 2869–2876. doi: 10.1016/j.egypro.2011.02.193
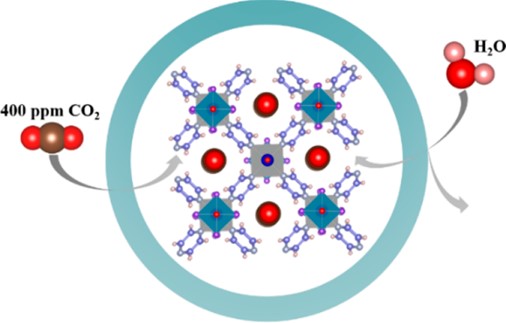
Downloads
Published
How to Cite
Issue
Section
License
Copyright (c) 2023 Jianfei Zheng, Xiaoping Chen, Jiliang Ma

This work is licensed under a Creative Commons Attribution-NonCommercial 4.0 International License.