3D-printed stretchable conductive polymer composites with nano-carbon fillers for multifunctional applications
DOI:
https://doi.org/10.18686/cest.v1i2.84Keywords:
carbon-based materials; 3D printing technology; polymer composites; structural design; electronic devicesAbstract
Carbon nanomaterials are widely used as substrate materials to prepare stretchable conductive composites due to their good stability, strong conductivity, and low price. In response to the demand for optimizing the performance of composite materials, various manufacturing methods for preparing carbon nanomaterial-reinforced stretchable conductive composite materials have emerged. Among them, 3D printing technology has the advantages of flexible processes and excellent product performance and has received widespread attention. This review focuses on the research progress of adding carbon nanomaterials as reinforcing phases to polymer materials using 3D printing technology. The application prospects of conductive polymer composites based on nanocarbon fillers in aerospace, energy storage, biomedicine, and other fields are prospected.
References
Cao D, Xing Y, Tantratian K, et al. 3D printed high-performance lithium metal microbatteries enabled by nanocellulose. Advanced Materials 2019; 31(14): 1807313. doi: 10.1002/adma.201807313
Chen X. Making electrodes stretchable. Small Methods 2017; 1(4): 1600029. doi: 10.1002/smtd.201600029
Lv Z, Li W, Yang L, et al. Custom-made electrochemical energy storage devices. ACS Energy Letters 2019; 4(2): 606–614. doi: 10.1021/acsenergylett.8b02408
Zhao C, Wang R, Fang B, et al. Boosting the lithium storage properties of a flexible Li4Ti5O12/graphene fiber anode via a 3D printing assembly strategy. Batteries 2023; 9(10): 493. doi: 10.3390/batteries9100493
Wang C, Xia K, Wang H, et al. Advanced carbon for flexible and wearable electronics. Advanced Materials 2019; 31(9): 1801072. doi: 10.1002/adma.201801072
Bokobza L. Mechanical and electrical properties of elastomer nanocomposites based on different carbon nanomaterials. C—Journal of Carbon Research 2017; 3(2): 10. doi: 10.3390/c3020010
Mondal S, Khastgir D. Elastomer reinforcement by graphene nanoplatelets and synergistic improvements of electrical and mechanical properties of composites by hybrid nano fillers of graphene-carbon black & graphene-MWCNT. Composites Part A: Applied Science and Manufacturing 2017; 102: 154–165. doi: 10.1016/j.compositesa.2017.08.003
Ryan KR, Down MP, Hurst NJ, et al. Additive manufacturing (3D printing) of electrically conductive polymers and polymer nanocomposites and their applications. eScience 2022; 2(4): 365–381. doi: 10.1016/j.esci.2022.07.003
Huang A, Ma Y, Peng J, et al. Tailoring the structure of silicon-based materials for lithium-ion batteries via electrospinning technology. eScience 2021; 1(2): 141–162. doi: 10.1016/j.esci.2021.11.006
Wang Z, Gao W, Zhang Q, et al. 3D-printed graphene/polydimethylsiloxane composites for stretchable and strain-insensitive temperature sensors. ACS Applied Materials & Interfaces 2019; 11(1): 1344–1352. doi: 10.1021/acsami.8b16139
Mo R, Rooney D, Sun K, Yang HY. 3D nitrogen-doped graphene foam with encapsulated germanium/nitrogen-doped graphene yolk-shell nanoarchitecture for high-performance flexible Li-ion battery. Nature Communications 2017; 8(1): 13949. doi: 10.1038/ncomms13949
Zhao C, Liang H, Wang R, et al. Recent advances in high value-added carbon materials prepared from carbon dioxide for energy storage applications. Carbon Capture Science & Technology 2023; 9: 100144. doi: 10.1016/j.ccst.2023.100144
de Leon AC, Chen Q, Palaganas NB, et al. High performance polymer nanocomposites for additive manufacturing applications. Reactive and Functional Polymers 2016; 103: 141–155. doi: 10.1016/j.reactfunctpolym.2016.04.010
Song WJ, Lee S, Song G, Park S. Stretchable aqueous batteries: Progress and prospects. ACS Energy Letters 2019; 4(1): 177–186. doi: 10.1021/acsenergylett.8b02053
Song Z, Ma T, Tang R, et al. Origami lithium-ion batteries. Nature Communications 2014; 5(1): 3140. doi: 10.1038/ncomms4140
Bao Y, Zhang XY, Zhang X, et al. Free-standing and flexible limntio4/carbon nanotube cathodes for high performance lithium ion batteries. Journal of Power Sources 2016; 321: 120–125. doi: 10.1016/j.jpowsour.2016.04.121
Fu KK, Cheng J, Li T, Hu L. Flexible batteries: From mechanics to devices. ACS Energy Letters 2016; 1(5): 1065–1079. doi: 10.1021/acsenergylett.6b00401
Bao Y, Hong G, Chen Y, et al. Customized kirigami electrodes for flexible and deformable lithium-ion batteries. ACS Applied Materials & Interfaces 2020; 12(1): 780–788. doi: 10.1021/acsami.9b18232
Storck JL, Ehrmann G, Uthoff J, Diestelhorst E. Investigating inexpensive polymeric 3D printed materials under extreme thermal conditions. Materials Futures 2022; 1(1): 015001. doi: 10.1088/2752-5724/ac4beb
de Castro Motta J, Qaderi S, Farina I, et al. Experimental characterization and mechanical modeling of additively manufactured TPU components of innovative seismic isolators. Acta Mechanica 2022. doi: 10.1007/s00707-022-03447-5
Buchanan C, Gardner L. Metal 3D printing in construction: A review of methods, research, applications, opportunities and challenges. Engineering Structures 2019; 180: 332–348. doi: 10.1016/j.engstruct.2018.11.045
Zhu C, Liu T, Qian F, et al. 3D printed functional nanomaterials for electrochemical energy storage. Nano Today 2017; 15: 107–120. doi: 10.1016/j.nantod.2017.06.007
Zhang F, Wei M, Viswanathan VV, et al. 3D printing technologies for electrochemical energy storage. Nano Energy 2017; 40: 418–431. doi: 10.1016/j.nanoen.2017.08.037
Sousa RE, Costa CM, Lanceros-Méndez S. Advances and future challenges in printed batteries. ChemSusChem 2015; 8(21): 3539–3555. doi: 10.1002/cssc.201500657
Tian X, Jin J, Yuan S, et al. Emerging 3D-printed electrochemical energy storage devices: A critical review. Advanced Energy Materials 2017; 7(17): 1700127. doi: 10.1002/aenm.201700127
Guo W, Wang X, Yang C, et al. Microfluidic 3D printing polyhydroxyalkanoates-based bionic skin for wound healing. Materials Futures 2022; 1: 015401. doi: 10.1088/2752-5724/ac446b
Park S, Shou W, Makatura L, et al. 3D printing of polymer composites: Materials, processes, and applications. Matter 2022; 5(1): 43–76. doi: 10.1016/j.matt.2021.10.018
de Leon AC, Rodier BJ, Bajamundi C, et al. Plastic metal-free electric motor by 3D printing of graphene-polyamide powder. ACS Applied Energy Materials 2018; 1(4): 1726–1733. doi: 10.1021/acsaem.8b00240
Hall A, Kong GX, Karanassios V. Detectors and light-sources for optical spectrometry: From a 3D-printed light-source to a self-powered sensor fabricated on a flexible polymeric substrate, and from there on to an IoT-enabled “smart” system. In: Proceedings of the 2019 IEEE International Conference on Flexible and Printable Sensors and Systems (FLEPS); 8–10 July 2019; Glasgow, UK. pp. 1–3. doi:10.1109/fleps.2019.8792321
Kurra N, Jiang Q, Nayak P, Alshareef HN. Laser-derived graphene: A three-dimensional printed graphene electrode and its emerging applications. Nano Today 2019; 24: 81–102. doi: 10.1016/j.nantod.2018.12.003
Li C, Cheng J, He Y, et al. Polyelectrolyte elastomer-based ionotronic sensors with multi-mode sensing capabilities via multi-material 3D printing. Nature Communications 2023; 14(1): 4853. doi: 10.1038/s41467-023-40583-5
Li K, Liang M, Wang H, et al. 3D mxene architectures for efficient energy storage and conversion. Advanced Functional Materials 2020; 30(47): 2000842. doi: 10.1002/adfm.202000842
Lyu Z, Lim GJH, Koh JJ, et al. Design and manufacture of 3D-printed batteries. Joule 2021; 5(1): 89–114. doi: 10.1016/j.joule.2020.11.010
Park J, Kim JK, Park SA, et al. 3D-printed biodegradable polymeric stent integrated with a battery-less pressure sensor for biomedical applications. In: Proceedings of the 2017 19th International Conference on Solid-State Sensors, Actuators and Microsystems (TRANSDUCERS); 18–22 June 2017; Kaohsiung, Taiwan. pp. 14–50. doi: 10.1109/transducers.2017.7993984
Ngo TD, Kashani A, Imbalzano G, et al. Additive manufacturing (3D printing): A review of materials, methods, applications and challenges. Composites Part B: Engineering 2018; 143: 172–196. doi: 10.1016/j.compositesb.2018.02.012
Zhu Y, Murali S, Cai W, et al. Graphene and graphene oxide: Synthesis, properties, and applications. Advanced Materials 2010; 22(35): 3906–3024. doi: 10.1002/adma.201001068
Unwin PR, Güell AG, Zhang G. Nanoscale electrochemistry of sp2 carbon materials: From graphite and graphene to carbon nanotubes. Accounts of Chemical Research 2016; 49(9): 2041–2408. doi: 10.1021/acs.accounts.6b00301
Smith M. New developments in carbon fiber. Reinforced Plastics 2018; 62(5): 266–269. doi: 10.1016/j.repl.2017.07.004
Fu X, Xu L, Li J, et al. Flexible solar cells based on carbon nanomaterials. Carbon 2018; 139: 1063–1073. doi: 10.1016/j.carbon.2018.08.017
Bhagavatheswaran ES, Parsekar M, Das A, et al. Construction of an interconnected nanostructured carbon black network: Development of highly stretchable and robust elastomeric conductors. The Journal of Physical Chemistry C 2015; 119(37): 21723–21731. doi: 10.1021/acs.jpcc.5b06629
Niu XZ, Peng SL, Liu LY, et al. Characterizing and patterning of PDMS-based conducting composites. Advanced Materials 2007; 19(18): 2682–2686. doi: 10.1002/adma.200602515
Song WJ, Park J, Kim DH, et al. Jabuticaba-inspired hybrid carbon filler/polymer electrode for use in highly stretchable aqueous Li-ion batteries. Advanced Energy Materials 2018; 8(10): 1702478. doi: 10.1002/aenm.201702478
Shin MK, Oh J, Lima M, et al. Elastomeric conductive composites based on carbon nanotube forests. Advanced Materials 2010; 22(24): 2663–2667. doi: 10.1002/adma.200904270
Sekitani T, Nakajima H, Maeda H, et al. Stretchable active-matrix organic light-emitting diode display using printable elastic conductors. Nature Materials 2009; 8(6): 494–499. doi: 10.1038/nmat2459
Liu Z, Qian Z, Song J, Zhang Y. Conducting and stretchable composites using sandwiched graphene-carbon nanotube hybrids and styrene-butadiene rubber. Carbon 2019; 149: 181–189. doi: 10.1016/j.carbon.2019.04.037
Gao N, Fang X. Synthesis and development of graphene—Inorganic semiconductor nanocomposites. Chemical Reviews 2015; 115(16): 8294–83343. doi: 10.1021/cr400607y
Chen Z, Ren W, Gao L, et al. Three-dimensional flexible and conductive interconnected graphene networks grown by chemical vapour deposition. Nature Materials 2011; 10(6): 424–428. doi: 10.1038/nmat3001
Wang Z, Liu X, Shen X, et al. An ultralight graphene honeycomb sandwich for stretchable light-emitting displays. Advanced Functional Materials 2018; 28(19): 1707043. doi: 10.1002/adfm.201707043
Sun F, Tian M, Sun X, et al. Stretchable conductive fibers of ultrahigh tensile strain and stable conductance enabled by a worm-shaped graphene microlayer. Nano Letters 2019; 19(9): 6592–6599. doi: 10.1021/acs.nanolett.9b02862
Praveena BA, Lokesh N, Buradi A, et al. A comprehensive review of emerging additive manufacturing (3D printing technology): Methods, materials, applications, challenges, trends and future potential. Materials Today: Proceedings 2022; 52(Part 3): 1309–1313. doi: 10.1016/J.MATPR.2021.11.059
Kristiawan RB, Imaduddin F, Ariawan D, et al. A review on the fused deposition modeling (FDM) 3D printing: Filament processing, materials, and printing parameters. Open Engineering 2021; 11(1): 639–649. doi: 10.1515/ENG-2021-0063
Pervaiz S, Qureshi TA, Kashwani G, Kannan S. 3D printing of fiber-reinforced plastic composites using fused deposition modeling: A status review. Materials 2021; 14(16): 4520. doi: 10.3390/MA14164520
Wei X, Li D, Jiang W, et al. 3D printable graphene composite. Scientific Reports 2015; 5(1): 11181. doi: 10.1038/srep11181
Zhu D, Ren Y, Liao G, et al. Thermal and mechanical properties of polyamide 12/graphene nanoplatelets nanocomposites and parts fabricated by fused deposition modeling. Journal of Applied Polymer Science 2017; 134(39): 45332. doi: 10.1002/app.45332
Saadi MASR, Maguire A, Pottackal NT, et al. Direct ink writing: A 3D printing technology for diverse materials. Advanced Materials 2022; 34(28): 2108855. doi: 10.1002/ADMA.202108855
Singh M, Haverinen HM, Dhagat P, Jabbour GE. Inkjet printing—Process and its applications. Advanced Materials 2010; 22(6): 673–685. doi: 10.1002/adma.200901141
Lim S, Kang B, Kwak D, et al. Inkjet-printed reduced graphene oxide/poly(vinyl alcohol) composite electrodes for flexible transparent organic field-effect transistors. The Journal of Physical Chemistry C 2012; 116(13): 7520–7525. doi: 10.1021/jp203441e
García-Tuñon E, Barg S, Franco J, et al. Printing in three dimensions with graphene. Advanced Materials 2015; 27(10): 1688–1693. doi: 10.1002/adma.201405046
Uçak N, Çiçek A, Aslantas K. Machinability of 3D printed metallic materials fabricated by selective laser melting and electron beam melting: A review. Journal of Manufacturing Processes 2022; 80: 414–457. doi: 10.1016/J.JMAPRO.2022.06.023
Acord KA, Dupuy AD, Scipioni Bertoli U, et al. Morphology, microstructure, and phase states in selective laser sintered lithium ion battery cathodes. Journal of Materials Processing Technology 2021; 288: 116827. doi: 10.1016/J.JMATPROTEC.2020.116827
Zhou X, Nowicki M, Cui H, et al. 3D bioprinted graphene oxide-incorporated matrix for promoting chondrogenic differentiation of human bone marrow mesenchymal stem cells. Carbon 2017; 116: 615–624. doi: 10.1016/j.carbon.2017.02.049
Pagac M, Hajnys J, Ma QP, et al. A review of vat photopolymerization technology: Materials, applications, challenges, and future trends of 3D printing. Polymers 2021; 13(4): 598. doi: 10.3390/POLYM13040598
Costa BMDC, Griveau S, Bedioui F, et al. Stereolithography based 3D-printed microfluidic device with integrated electrochemical detection. Electrochimica Acta 2022; 407: 139888. doi: 10.1016/J.ELECTACTA.2022.139888
Li C, Du J, Gao Y, et al. Stereolithography of 3D sustainable metal electrodes towards high‐performance nickel iron battery. Advanced Functional Materials 2022; 32(40): 2205317. doi: 10.1002/ADFM.202205317
Gaikwad S, Tate JS, Theodoropoulou N, Koo JH. Electrical and mechanical properties of PA11 blended with nanographene platelets using industrial twin-screw extruder for selective laser sintering. Journal of Composite Materials 2013; 47(23): 2973–2986. doi: 10.1177/0021998312460560
Shuai C, Feng P, Gao C, et al. Graphene oxide reinforced poly(vinyl alcohol): Nanocomposite scaffolds for tissue engineering applications. RSC Advances 2015; 5: 25416–25423. doi: 10.1039/C4RA16702C
Zhai F, Feng Y, Li Z, et al. 4D-printed untethered self-propelling soft robot with tactile perception: Rolling, racing, and exploring. Matter 2021; 4(10): 3313–3326. doi: 10.1016/J.MATT.2021.08.014
Yu Y, Feng Y, Liu F, et al. Carbon dots-based ultrastretchable and conductive hydrogels for high-performance tactile sensors and self-powered electronic skin. Small 2023; 19(31): 2204365. doi: 10.1002/SMLL.202204365
Zhu Y, Tang T, Zhao S, et al. Recent advancements and applications in 3D printing of functional optics. Additive Manufacturing 2022; 52: 102682. doi: 10.1016/J.ADDMA.2022.102682
Jiang Y, Islam MN, He R, et al. Recent advances in 3D printed sensors: Materials, design, and manufacturing. Advanced Materials Technologies 2023; 8(2): 2200492. doi: 10.1002/ADMT.202200492
Tan HW, Choong YYC, Kuo CN, et al. 3D printed electronics: Processes, materials and future trends. Progress in Materials Science 2022; 127: 100945. doi: 10.1016/J.PMATSCI.2022.100945
Zhang F, Feng Y, Feng W. Three-dimensional interconnected networks for thermally conductive polymer composites: Design, preparation, properties, and mechanisms. Materials Science and Engineering: R: Reports 2020; 142: 100580. doi: 10.1016/J.MSER.2020.100580
Li Z, Wang L, Li Y, et al. Carbon-based functional nanomaterials: Preparation, properties and applications. Composites Science and Technology 2019; 179: 10–40. doi: 10.1016/J.COMPSCITECH.2019.04.028
Zhang D, Chi B, Li B, et al. Fabrication of highly conductive graphene flexible circuits by 3D printing. Synthetic Metals 2016; 217: 79–86. doi: 10.1016/j.synthmet.2016.03.014
Liu H, Zhang H, Han W, et al. 3D printed flexible strain sensors: From printing to devices and signals. Advanced Materials 2021; 33(8): 2004782. doi: 10.1002/ADMA.202004782
Schwierz F. Graphene transistors. Nature Nanotechnology 2010;5(7): 487–496. doi: 10.1038/nnano.2010.89
Xiang L, Wang Z, Liu Z, et al. Inkjet-printed flexible biosensor based on graphene field effect transistor. IEEE Sensors Journal 2016; 16(23): 8359–8364. doi: 10.1109/JSEN.2016.2608719
Huang L, Huang Y, Liang J, et al. Graphene-based conducting inks for direct inkjet printing of flexible conductive patterns and their applications in electric circuits and chemical sensors. Nano Research 2011; 4(7): 675–684. doi: 10.1007/s12274-011-0123-z
Willian MD, Michaela E, Mae CH, et al. A comprehensive review on the application of 3D printing in the aerospace industry. Key Engineering Materials 2022; 913: 27–34. doi: 10.4028/p-94a9zb
Mohanavel V, Ashraff Ali KS, Ranganathan K, et al. The roles and applications of additive manufacturing in the aerospace and automobile sector. Materials Today: Proceedings 2021; 47: 405–409. doi: 10.1016/J.MATPR.2021.04.596
Sugiyama K, Matsuzaki R, Ueda M, et al. 3D printing of composite sandwich structures using continuous carbon fiber and fiber tension. Composites Part A: Applied Science and Manufacturing 2018; 113: 114–121. doi: 10.1016/J.COMPOSITESA.2018.07.029
Sai Saran O, Prudhvidhar Reddy A, Chaturya L, Pavan Kumar M. 3D printing of composite materials: A short review. Materials Today: Proceedings 2022; 64: 615–619. doi: 10.1016/J.MATPR.2022.05.144
Wang X, Jin J, Song M. An investigation of the mechanism of graphene toughening epoxy. Carbon 2013; 65: 324–333. doi: 10.1016/j.carbon.2013.08.032
Verma M, Verma P, Dhawan SK, Choudhary V. Tailored graphene based polyurethane composites for efficient electrostatic dissipation and electromagnetic interference shielding applications. RSC Advances 2015; 5(118): 97349–97358. doi: 10.1039/C5RA17276D
Singh AP, Garg P, Alam F, et al. Phenolic resin-based composite sheets filled with mixtures of reduced graphene oxide, γ-Fe2O3 and carbon fibers for excellent electromagnetic interference shielding in the X-band. Carbon 2012; 50(10): 3868–3875. doi: 10.1016/j.carbon.2012.04.030
Fu K, Wang Y, Yan C, et al. Graphene oxide-based electrode inks for 3D-printed lithium-ion batteries. Advanced Materials 2016; 28(13): 2587–2594. doi: 10.1002/adma.201505391
Rocha VG, García-Tuñón E, Botas C, et al. Multimaterial 3D printing of graphene-based electrodes for electrochemical energy storage using thermoresponsive inks. ACS Applied Materials&Interfaces 2017; 9(42): 37136–37145. doi: 10.1021/acsami.7b10285
Shen K, Mei H, Li B, et al. 3D printing sulfur copolymer-graphene architectures for Li-S batteries. Advanced Energy Materials 2018; 8(4): 1701527. doi: 10.1002/aenm.201701527
Huang K, Yang J, Dong S, et al. Anisotropy of graphene scaffolds assembled by three-dimensional printing. Carbon 2018; 130: 1–10. doi: 10.1016/j.carbon.2017.12.120
Vernardou D, Vasilopoulos KC, Kenanakis G. 3D printed graphene-based electrodes with high electrochemical performance. Applied Physics A 2017; 123: 623. doi: 10.1007/s00339-017-1238-1
Fan D, Li Y, Wang X, et al. Progressive 3D printing technology and its application in medical materials. Frontiers in Pharmacology 2020; 11: 516624. doi: 10.3389/FPHAR.2020.00122/BIBTEX
Al-Dulimi Z, Wallis M, Tan DK, et al. 3D printing technology as innovative solutions for biomedical applications. Drug Discovery Today 2021; 26(2): 360–383. doi: 10.1016/J.DRUDIS.2020.11.013
Chadha U, Abrol A, Vora NP, et al. Performance evaluation of 3D printing technologies: A review, recent advances, current challenges, and future directions. Progress in Additive Manufacturing 2022; 7(5): 853–886. doi: 10.1007/S40964-021-00257-4
Mallakpour S, Tabesh F, Hussain CM. 3D and 4D printing: From innovation to evolution. Advances in Colloid and Interface Science 2021; 294: 102482. doi: 10.1016/J.CIS.2021.102482
Zhang L, Forgham H, Shen A, et al. Nanomaterial integrated 3D printing for biomedical applications. Journal of Materials Chemistry B 2022; 10(37): 7473–7490. doi: 10.1039/D2TB00931E
Kantaros A. 3D printing in regenerative medicine: Technologies and resources utilized. International Journal of Molecular Sciences 2022; 23(23): 14621. doi: 10.3390/IJMS232314621
Pavan Kalyan BG, Kumar L. 3D printing: Applications in tissue engineering, medical devices, and drug delivery. AAPS PharmSciTech 2022; 23(4): 1–20. doi: 10.1208/S12249-022-02242-8
Kalkal A, Kumar S, Kumar P, et al. Recent advances in 3D printing technologies for wearable (bio)sensors. Additive Manufacturing 2021; 46: 102088. doi: 10.1016/J.ADDMA.2021.102088
Bozkurt Y, Karayel E. 3D printing technology; methods, biomedical applications, future opportunities and trends. Journal of Materials Research and Technology 2021; 14: 1430–1450. doi: 10.1016/J.JMRT.2021.07.050
Wang W, Caetano G, Ambler WS, et al. Enhancing the hydrophilicity and cell attachment of 3D printed PCL/graphene scaffolds for bone tissue engineering. Materials (Basel) 2016; 9(12): 992. doi: 10.3390/ma9120992
Cheng Z, Landish B, Chi Z, et al. 3D printing hydrogel with graphene oxide is functional in cartilage protection by influencing the signal pathway of Rank/Rankl/OPG. Materials Science and Engineering: C 2018; 82: 244–252. doi: 10.1016/j.msec.2017.08.069
Chen Q, Mangadlao JD, Wallat J, et al. 3D printing biocompatible polyurethane/poly(lactic acid)/graphene oxide nanocomposites: Anisotropic properties. ACS Applied Materials & Interfaces 2017; 9(4): 4015–4023. doi: 10.1021/acsami.6b11793
Sayyar S, Bjorninen M, Haimi S, et al. UV cross-linkable graphene/poly(trimethylene carbonate) composites for 3D printing of electrically conductive scaffolds. ACS Applied Materials & Interfaces 2016; 8(46): 31916–31925. doi: 10.1021/acsami.6b09962
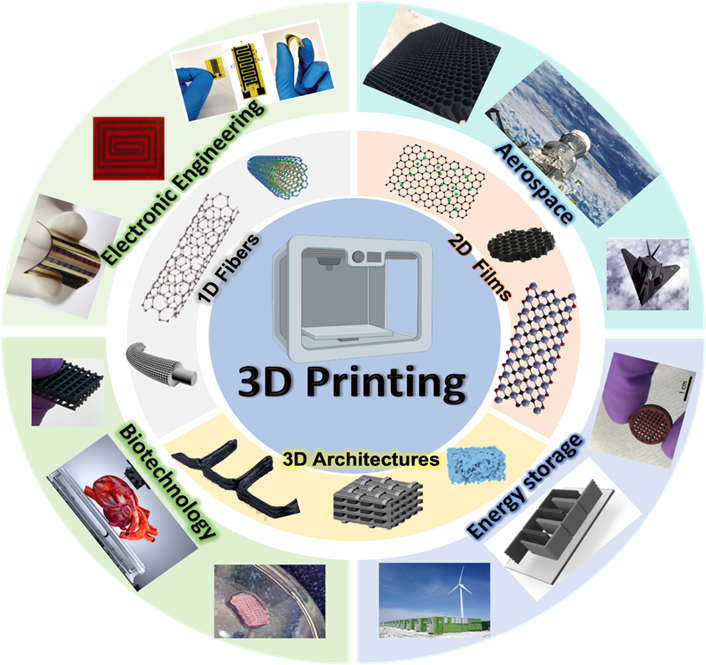
Downloads
Published
How to Cite
Issue
Section
License
Copyright (c) 2023 Chenpeng Zhao, Ruqing Li, Biao Fang, Rui Wang, Han Liang, Lei Wang, Ruilin Wu, Yunan Wei, Zhangyuan Wang, Zhipeng Su, Runwei Mo

This work is licensed under a Creative Commons Attribution-NonCommercial 4.0 International License.