Flue gas CO2 supply methods for microalgae utilization: A review
DOI:
https://doi.org/10.18686/cest.v1i2.78Abstract
The potential for utilizing flue gas as a carbon source in microalgal cultivation holds great promise. Incorporating flue gas as a carbon source into microalgae culture processes can accelerate the growth rate of microalgae, consequently enhancing the overall economic viability of the integrated process. There are two key sources of flue gas to consider: flue gas from coal-fired power plants, characterized by a CO2 concentration of 12%–15% w/w, and flue gas from coal chemical processes, boasting a CO2 concentration of 90%–99% w/w. Additionally, the choice between an open or sealed microalgae culture system can also influence economic efficiency. Thus, there are four distinct microalgal cultivation routes to assess: in-situ open systems, off-situ open systems, in-situ sealed systems, and off-situ sealed systems. The incorporation of flue gas as a carbon source in microalgae cultivation demonstrates significant potential for reducing both environmental impact and costs, rendering it a highly promising and sustainable approach for economically efficient microalgae cultivation. In this review, the in-situ open route is recommended for systems with a high concentration of flue gas CO2 with the target product of low-margin commodities, while the off-situ sealed route is suitable for systems with low concentration of flue gas CO2 with the target product of high-value-added products.
References
Lu G, Wang Z, Bhatti UH, et al. Recent progress in carbon dioxide capture technologies: A review. Clean Energy Science and Technology 2023; 1(1). doi: 10.18686/cest.v1i1.32
Zhang S, Liu Z. Advances in the biological fixation of carbon dioxide by microalgae. Journal of Chemical Technology & Biotechnology 2021; 96(6): 1475–1495. doi: 10.1002/jctb.6714
Magalhães IB, Ferreira J, de Siqueira Castro J, et al. Agro-industrial wastewater-grown microalgae: A techno-environmental assessment of open and closed systems. Science of The Total Environment 2022; 834: 155282. doi: 10.1016/j.scitotenv.2022.155282
Clarens AF, Resurreccion EP, White MA, et al. Environmental life cycle comparison of algae to other bioenergy feedstocks. Environmental Science & Technology 2010; 44(5): 1813–1819. doi: 10.1021/es902838n
Ruiz J, Olivieri G, de Vree J, et al. Towards industrial products from microalgae. Energy & Environmental Science 2016; 9(10): 3036–3043. doi: 10.1039/c6ee01493c
De Assis TC, Calijuri ML, Assemany PP, et al. Using atmospheric emissions as CO2 source in the cultivation of microalgae: Productivity and economic viability. Journal of Cleaner Production 2019; 215: 1160–1169. doi: 10.1016/j.jclepro.2019.01.093
Zhu Y, Cheng J, Zhang Z, et al. Mutation of Arthrospira platensis by gamma irradiation to promote phenol tolerance and CO2 fixation for coal-chemical flue gas reduction. Journal of CO2 Utilization 2020; 38: 252–261. doi: 10.1016/j.jcou.2020.02.003
Zhao Q, Jin G, Liu Q, et al. Tolerance comparison among selected spirulina strains cultured under high carbon dioxide and coal power plant flue gas supplements. Journal of Ocean University of China 2021; 20(6): 1567–1577. doi: 10.1007/s11802-021-4783-3
Mondal M, Ghosh A, Gayen K, et al. Carbon dioxide bio-fixation by Chlorella sp. BTA 9031 towards biomass and lipid production: Optimization using central composite design approach. Journal of CO2 Utilization 2017; 22: 317–329. doi: 10.1016/j.jcou.2017.10.008
Sung YJ, Lee JS, Yoon HK, et al. Outdoor cultivation of microalgae in a coal-fired power plant for conversion of flue gas CO2 into microalgal direct combustion fuels. Systems Microbiology and Biomanufacturing 2020; 1(1): 90–99. doi: 10.1007/s43393-020-00007-7
Sarat Chandra T, Deepak RS, Maneesh Kumar M, et al. Evaluation of indigenous fresh water microalga Scenedesmus obtusus for feed and fuel applications: Effect of carbon dioxide, light and nutrient sources on growth and biochemical characteristics. Bioresource Technology 2016; 207: 430–439. doi: 10.1016/j.biortech.2016.01.044
Kim GY, Heo J, Kim HS, et al. Bicarbonate-based cultivation of Dunaliella salina for enhancing carbon utilization efficiency. Bioresource Technology 2017; 237: 72–77. doi: 10.1016/j.biortech.2017.04.009
Zhu C, Chen S, Ji Y, et al. Progress toward a bicarbonate-based microalgae production system. Trends in Biotechnology 2022; 40(2): 180–193. doi: 10.1016/j.tibtech.2021.06.005
Zhu C, Zhang R, Cheng L, et al. A recycling culture of Neochloris oleoabundans in a bicarbonate-based integrated carbon capture and algae production system with harvesting by auto-flocculation. Biotechnology for Biofuels 2018; 11(1). doi: 10.1186/s13068-018-1197-6
Acién Fernández FG, Fernández Sevilla JM, Molina Grima E. Costs analysis of microalgae production. In: Pandey A, Chang JS, Soccol CR, et al (editors). Biofuels from Algae, 2nd ed. Elsevier; 2019. pp. 551–566. doi: 10.1016/b978-0-444-64192-2.00021-4
Shekh A, Sharma A, Schenk PM, et al. Microalgae cultivation: Photobioreactors, CO2 utilization, and value‐added products of industrial importance. Journal of Chemical Technology & Biotechnology 2021; 97(5): 1064–1085. doi: 10.1002/jctb.6902
Acién Fernández FG, Reis A, Wijffels RH, et al. The role of microalgae in the bioeconomy. New Biotechnology 2021; 61: 99–107. doi: 10.1016/j.nbt.2020.11.011
Morales-Amaral MDM, Gómez-Serrano C, Acién FG, et al. Outdoor production of Scenedesmus sp. in thin-layer and raceway reactors using centrate from anaerobic digestion as the sole nutrient source. Algal Research 2015; 12: 99–108. doi: 10.1016/j.algal.2015.08.020
Venancio HC, Cella H, Lopes RG, et al. Surface-to-volume ratio influence on the growth of Scenedesmus obliquus in a thin-layer cascade system. Journal of Applied Phycology 2020; 32(2): 821–829. doi: 10.1007/s10811-020-02036-0
Villaró S, Sánchez-Zurano A, Ciardi M, et al. Production of microalgae using pilot-scale thin-layer cascade photobioreactors: Effect of water type on biomass composition. Biomass and Bioenergy 2022; 163: 106534. doi: 10.1016/j.biombioe.2022.106534
Zhu B, Shen H, Li Y, et al. Large-scale cultivation of spirulina for biological CO2 mitigation in open raceway ponds using purified CO2 from a coal chemical flue gas. Frontiers in Bioengineering and Biotechnology 2020; 7. doi: 10.3389/fbioe.2019.00441
Day JG, Gong Y, Hu Q. Microzooplanktonic grazers—A potentially devastating threat to the commercial success of microalgal mass culture. Algal Research 2017; 27: 356–365. doi: 10.1016/j.algal.2017.08.024
Rasheed R, Thaher M, Younes N, et al. Solar cultivation of microalgae in a desert environment for the development of techno-functional feed ingredients for aquaculture in Qatar. Science of The Total Environment 2022; 835: 155538. doi: 10.1016/j.scitotenv.2022.155538
Mohan N, Rao PH, Boopathy AB, et al. A sustainable process train for a marine microalga-mediated biomass production and CO2 capture: A pilot-scale cultivation of Nannochloropsis salina in open raceway ponds and harvesting through electropreciflocculation. Renewable Energy 2021; 173: 263–272. doi: 10.1016/j.renene.2021.03.147
Vadlamani A, Pendyala B, Viamajala S, et al. High productivity cultivation of microalgae without concentrated CO2 input. ACS Sustainable Chemistry & Engineering 2018; 7(2): 1933–1943. doi: 10.1021/acssuschemeng.8b04094
Giostri A, Binotti M, Macchi E. Microalgae cofiring in coal power plants: Innovative system layout and energy analysis. Renewable Energy 2016; 95: 449–464. doi: 10.1016/j.renene.2016.04.033
Magalhães IB, Ferreira J, de Siqueira Castro J, et al. Technologies for improving microalgae biomass production coupled to effluent treatment: A life cycle approach. Algal Research 2021; 57: 102346. doi: 10.1016/j.algal.2021.102346
Acedo M, Gonzalez Cena JR, Kiehlbaugh KM, et al. Coupling carbon capture from a power plant with semi-automated open raceway ponds for microalgae cultivation. Journal of Visualized Experiments 2020; 162. doi: 10.3791/61498-v
Zhang RL, Wang JH, Cheng LY, et al. Selection of microalgae strains for bicarbonate-based integrated carbon capture and algal production system to produce lipid. International Journal of Green Energy 2019; 16(11): 825–833. doi: 10.1080/15435075.2019.1641103
Sampathkumar SJ, Gothandam KM. Sodium bicarbonate augmentation enhances lutein biosynthesis in green microalgae Chlorella pyrenoidosa. Biocatalysis and Agricultural Biotechnology 2019; 22: 101406. doi: 10.1016/j.bcab.2019.101406
Xia B, Chen B, Sun X, et al. Interaction of TiO2 nanoparticles with the marine microalga Nitzschia closterium: Growth inhibition, oxidative stress and internalization. Science of The Total Environment 2015; 508: 525–533. doi: 10.1016/j.scitotenv.2014.11.066
Zhu C, Zhai X, Jia J, et al. Seawater desalination concentrate for cultivation of Dunaliella salina with floating photobioreactor to produce β-carotene. Algal Research 2018; 35: 319–324. doi: 10.1016/j.algal.2018.08.035
Costa JAV, Freitas BCB, Rosa GM, et al. Operational and economic aspects of Spirulina-based biorefinery. Bioresource Technology 2019; 292: 121946. doi: 10.1016/j.biortech.2019.121946
Borovkov AB, Gudvilovich IN, Avsiyan AL, et al. Light supply and mineral nutrition conditions as optimization factors for outdoor mass culture of carotenogenic microalga Dunaliella salina. Aquaculture Research 2021; 52(12): 6098–6106. doi: 10.1111/are.15471
Bartley ML, Boeing WJ, Dungan BN, et al. pH effects on growth and lipid accumulation of the biofuel microalgae Nannochloropsis salina and invading organisms. Journal of Applied Phycology 2013; 26(3): 1431–1437. doi: 10.1007/s10811-013-0177-2
Xu H, Lee U, Coleman AM, et al. Balancing water sustainability and productivity objectives in microalgae cultivation: Siting open ponds by considering seasonal water-stress impact using AWARE-US. Environmental Science & Technology 2020; 54(4): 2091–2102. doi: 10.1021/acs.est.9b05347
Venteris ER, Skaggs RL, Coleman AM, et al. A GIS cost model to assess the availability of freshwater, seawater, and saline groundwater for algal biofuel production in the United States. Environmental Science & Technology 2013; 47(9): 4840–4849. doi: 10.1021/es304135b
Yang J, Xu M, Zhang X, et al. Life-cycle analysis on biodiesel production from microalgae: Water footprint and nutrients balance. Bioresource Technology 2011; 102(1): 159–165. doi: 10.1016/j.biortech.2010.07.017
Villaró S, Morillas-España A, Acién G, et al. Optimisation of operational conditions during the production of arthrospira platensis using pilot-scale raceway reactors, protein extraction, and assessment of their techno-functional properties. Foods 2022; 11(15): 2341. doi: 10.3390/foods11152341
Polle JEW, Jin E, Ben-Amotz A. The alga Dunaliella revisited: Looking back and moving forward with model and production organisms. Algal Research 2020; 49: 101948. doi: 10.1016/j.algal.2020.101948
Fabris M, Abbriano RM, Pernice M, et al. Emerging technologies in algal biotechnology: Toward the establishment of a sustainable, algae-based bioeconomy. Frontiers in Plant Science 2020; 11. doi: 10.3389/fpls.2020.00279
Guo W, Cheng J, Ali KA, et al. Conversion of NaHCO3 to Na2CO3 with a growth of Arthrospira platensis cells in 660 m2 raceway ponds with a CO2 bicarbonation absorber. Microbial Biotechnology 2019; 13(2): 470–478. doi: 10.1111/1751-7915.13497
Eustance E, Badvipour S, Wray JT, et al. Biomass productivity of two Scenedesmus strains cultivated semi-continuously in outdoor raceway ponds and flat-panel photobioreactors. Journal of Applied Phycology 2015; 28(3): 1471–1483. doi: 10.1007/s10811-015-0710-6
Rajkumar R, Takriff MS, Veeramuthu A. Technical insights into carbon dioxide sequestration by microalgae: A biorefinery approach towards sustainable environment. Biomass Conversion and Biorefinery 2022. doi: 10.1007/s13399-022-02446-9
Chauton MS, Reitan KI, Norsker NH, et al. A techno-economic analysis of industrial production of marine microalgae as a source of EPA and DHA-rich raw material for aquafeed: Research challenges and possibilities. Aquaculture 2015; 436: 95–103. doi: 10.1016/j.aquaculture.2014.10.038
Vázquez-Romero B, Perales JA, Pereira H, et al. Techno-economic assessment of microalgae production, harvesting and drying for food, feed, cosmetics, and agriculture. Science of The Total Environment 2022; 837: 155742. doi: 10.1016/j.scitotenv.2022.155742
Schipper K, Al-Jabri HMSJ, Wijffels RH, et al. Techno-economics of algae production in the Arabian Peninsula. Bioresource Technology 2021; 331: 125043. doi: 10.1016/j.biortech.2021.125043
Norsker NH, Barbosa MJ, Vermuë MH, et al. Microalgal production—A close look at the economics. Biotechnology Advances 2011; 29(1): 24–27. doi: 10.1016/j.biotechadv.2010.08.005
Suzuki H, Hulatt CJ, Wijffels RH, et al. Correction to: Growth and LC-PUFA production of the cold-adapted microalga Koliella antarctica in photobioreactors. Journal of Applied Phycology 2018; 31(2): 999. doi: 10.1007/s10811-018-1646-4
Choi YY, Joun JM, Lee J, et al. Development of large-scale and economic pH control system for outdoor cultivation of microalgae Haematococcus pluvialis using industrial flue gas. Bioresource Technology 2017; 244: 1235–1244. doi: 10.1016/j.biortech.2017.05.147
Tredici MR, Rodolfi L, Biondi N, et al. Techno-economic analysis of microalgal biomass production in a 1-ha Green Wall Panel (GWP®) plant. Algal Research 2016; 19: 253–263. doi: 10.1016/j.algal.2016.09.005
Oostlander PC, van Houcke J, Wijffels RH, et al. Microalgae production cost in aquaculture hatcheries. Aquaculture 2020; 525: 735310. doi: 10.1016/j.aquaculture.2020.735310
López AR, Rodríguez SB, Vallejo RA, et al. Sustainable cultivation of Nannochloropsis gaditana microalgae in outdoor raceways using flue gases for a complete 2-year cycle: A circular economy challenge. Journal of Applied Phycology 2019; 31(3): 1515–1523. doi: 10.1007/s10811-018-1710-0
Ye Q, Cheng J, Liu S, et al. Improving light distribution and light/dark cycle of 900 L tangential spiral-flow column photobioreactors to promote CO2 fixation with Arthrospira sp. cells. Science of The Total Environment 2020; 720: 137611. doi: 10.1016/j.scitotenv.2020.137611
Choi SY, Sim SJ, Ko SC, et al. Scalable cultivation of engineered cyanobacteria for squalene production from industrial flue gas in a closed photobioreactor. Journal of Agricultural and Food Chemistry 2020; 68(37): 10050–10055. doi: 10.1021/acs.jafc.0c03133
Zhu C, Zhai X, Xi Y, et al. Progress on the development of floating photobioreactor for microalgae cultivation and its application potential. World Journal of Microbiology and Biotechnology 2019; 35(12). doi: 10.1007/s11274-019-2767-x
Zhu C, Xi Y, Zhai X, et al. Pilot outdoor cultivation of an extreme alkalihalophilic Trebouxiophyte in a floating photobioreactor using bicarbonate as carbon source. Journal of Cleaner Production 2021; 283: 124648. doi: 10.1016/j.jclepro.2020.124648
Huang JJ, Bunjamin G, Teo ES, et al. An enclosed rotating floating photobioreactor (RFP) powered by flowing water for mass cultivation of photosynthetic microalgae. Biotechnology for Biofuels 2016; 9(1). doi: 10.1186/s13068-016-0633-8
Cheng J, Yang Z, Huang Y, et al. Improving growth rate of microalgae in a 1191 m2 raceway pond to fix CO2 from flue gas in a coal-fired power plant. Bioresource Technology 2015; 190: 235–241. doi: 10.1016/j.biortech.2015.04.085
Mitra R, Das Gupta A, Kumar RR, et al. A cleaner and smarter way to achieve high microalgal biomass density coupled with facilitated self-flocculation by utilizing bicarbonate as a source of dissolved carbon dioxide. Journal of Cleaner Production 2023; 391: 136217. doi: 10.1016/j.jclepro.2023.136217
Eustance E, Lai YJS, Shesh T, et al. Improved CO2 utilization efficiency using membrane carbonation in outdoor raceways. Algal Research 2020; 51: 102070. doi: 10.1016/j.algal.2020.102070
Ding Y, Li X, Wang Z, et al. Ammonium bicarbonate supplementation as carbon source in alkaliphilic Spirulina mass culture. Aquaculture Research 2017; 48(9): 4886–4896. doi: 10.1111/are.13308
Soletto D, Binaghi L, Ferrari L, et al. Effects of carbon dioxide feeding rate and light intensity on the fed-batch pulse-feeding cultivation of Spirulina platensis in helical photobioreactor. Biochemical Engineering Journal 2008; 39(2): 369–375. doi: 10.1016/j.bej.2007.10.007
Huntley ME, Johnson ZI, Brown SL, et al. Demonstrated large-scale production of marine microalgae for fuels and feed. Algal Research 2015; 10: 249–265. doi: 10.1016/j.algal.2015.04.016
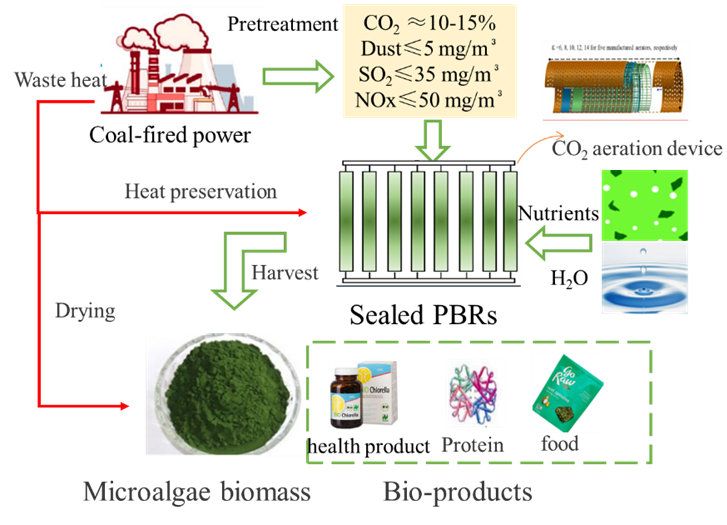
Downloads
Published
How to Cite
Issue
Section
License
Copyright (c) 2023 Xiaosu Yu, Wangbiao Guo, Zhan Hu, Pengcheng Li, Zhuowei (Amanda) Zhang, Jun Cheng, Chunfeng Song, Qing Ye

This work is licensed under a Creative Commons Attribution-NonCommercial 4.0 International License.