Redox flow battery technology development from perspective of patent applications: A review
DOI:
https://doi.org/10.18686/cest.v1i1.47Keywords:
redox flow batteries; ion exchange membranes; electrolytes; electrodes; bipolar platesAbstract
With the continuous increase in global energy consumption, the development and utilization of renewable energy become imperative. However, the intermittency and fluctuation of wind and solar power generation prevent direct grid integration, resulting in energy waste. As a large-scale electrochemical energy storage technology, redox flow batteries (RFBs) can effectively store renewable energy and smooth power output. In this paper, the development history of RFB technology in China is summarized by analyzing relevant patent application data and elaborating on the working principles, advantages and disadvantages of various RFBs, and their latest research progress. The technical challenges in current RFB research are analyzed and the application prospects of RFB commercialization are presented. The results showed that although RFB technology has made significant progress in China, it still faces issues, such as high battery cost and limited cycle life. To realize the efficient utilization of renewable energy and green low-carbon development, RFB technology needs continuous optimization and upgrade. This paper can provide references for the development of RFB technology.
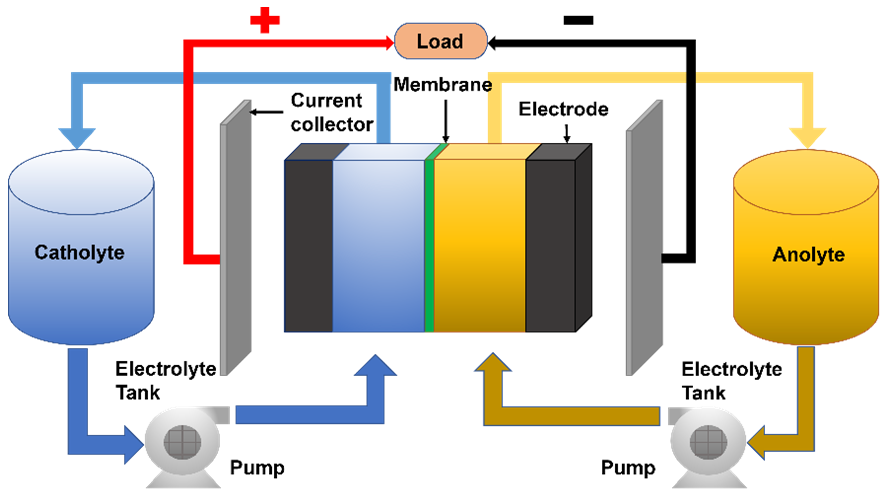
Downloads
Published
How to Cite
Issue
Section
License
Copyright (c) 2023 Wuyang Wang, Anle Mu, Bin Yang, Jiahui Wang, Yupeng Wang

This work is licensed under a Creative Commons Attribution-NonCommercial 4.0 International License.
References
1. Mu A, Liu H, Zhang M, Wang J. Theory and kinematics analysis of a novel variable speed constant frequency wind energy conversion system (Chinese). Journal of Mechanical Engineering 2008; (1): 195–198, 204.
2. Emmett RK, Roberts ME. Recent developments in alternative aqueous redox flow batteries for grid-scale energy storage. Journal of Power Sources 2021; 506: 230087. doi: 10.1016/j.jpowsour.2021.230087
3. Huang Z, Mu A, Wu L, et al. Comprehensive analysis of critical issues in all-vanadium redox flow battery. ACS Sustainable Chemistry & Engineering 2022; 10(24): 7786–7810. doi: 10.1021/acssuschemeng.2c01372
4. Kangro W. Method for Storing Electrical Energy. DE Patent 914,264, 28 June 1954.
5. Thaller LH. Electrically Rechargeable REDOX Flow Cell. U.S. Patent 3,996,064, 7 December 1976.
6. Xiao Y, Xiao K. High-Energy Static Vanadium Battery. CN Patent 1,507,103, 23 June 2004.
7. Li L. A 250 kWh long-duration advanced iron-chromium redox flow battery. In: The Electrochemical Society Meeting Abstracts, Proceedings of the 29th ECS Meeting with the 18th International Meeting on Chemical Sensors (IMCS); 30 May–3 June 2021; Online meeting. doi: 10.1149/MA2021-013222mtgabs
8. Flox C, Zhang C, Li Y. Redox flow battery as an emerging technology: Current status and research trends. Current Opinion in Chemical Engineering 2023; 39: 100880. doi: 10.1016/j.coche.2022.100880
9. Yao C, Zhang H, Liu T, et al. Cell architecture upswing based on catalyst coated membrane (CCM) for vanadium flow battery. Journal of Power Sources 2013; 237: 19–25. doi: 10.1016/j.jpowsour.2013.03.014
10. Sumitomo Corporation. Available online: https://www.sumitomocorp.com/en/jp (accessed on 20 March 2023).
11. LG Chem. Available online: https://www.lgchem.com/main/index (accessed on 20 March 2023).
12. Lotte Chemical. Homepage. Available online: https://www.lottechem.com/cn/index.do (accessed on 21 March 2023).
13. Division of Energy Storage. Available online: http://www.energystorage.dicp.ac.cn/ (accessed on 4 May 2023).
14. Rongke Energy Storage. Available online: http://www.rongkepower.com/?about/5.html (accessed on 25 March 2023).
15. State Grid. Available online: http://www.sgepri.sgcc.com.cn/ (accessed on 4 April 2023).
16. China Dongfang Electric Group Co., Ltd. Available online: https://www.dongfang.com/ (accessed on 4 April 2023).
17. 100-megawatt Dalian liquid flow battery energy storage peak-shaving power station connected to the grid for power generation. Available online: https://www.cas.cn/zkyzs/2022/11/369/kyjz/202211/t20221108_4854124.shtml (accessed on 8 April 2023).
18. Transcript of the press conference of the National Energy Administration in the first quarter of 2023. Available online: http://www.nea.gov.cn/2023-02/13/c_1310697149.htm (accessed on 11 September 2023).
19. Sánchez-Díez E, Ventosa E, Guarnieri M, et al. Redox flow batteries: Status and perspective towards sustainable stationary energy storage. Journal of Power Sources 2021; 481: 228804 doi: 10.1016/j.jpowsour.2020.228804
20. Fu H, Bao X, He M, et al. Defect-rich graphene skin modified carbon felt as a highly enhanced electrode for vanadium redox flow batteries. Journal of Power Sources 2023; 556: 232443. doi: 10.1016/j.jpowsour.2022.232443
21. Polaris wind power grid, Liaoning’s first all-vanadium flow battery energy storage power station connected to the grid. Available online: https://news.bjx.com.cn/html/20130304/420483.shtml (accessed on 2 May 2023).
22. The Dalian Institute of Chemical Physics, Chinese Academy of Sciences, and the all-vanadium redox flow battery energy storage technology research collective of our institute won the 2014 Chinese Academy of Sciences Outstanding Scientific and Technological Achievement Award. Available online: http://www.dicp.cas.cn/xwdt/zhxws/2015/201809/t20180930_5117290.html (accessed on 5 May 2023).
23. Liu T, Ge L, Zhang Y. Key technology progress and development trend of all-vanadium redox flow battery (Chinese). China Metallurgy 2023; 33(4): 1–8, 133. doi: 10.13228/j.boyuan.issn1006-9356.20221005
24. Gao L, Li Z, Zou Y, et al. A high-performance aqueous zinc-bromine static battery. Iscience 2020; 23(8): 101348. doi: 10.1016/j.isci.2020.101348
25. Shin K, Lee JH, Heo J, et al. Current status and challenges for practical flowless Zn-Br batteries. Current Opinion in Electrochemistry 2022; 32: 100898. doi: 10.1016/j.coelec.2021.100898
26. Lee Y, Yun D, Park J, et al. An organic imidazolium derivative additive inducing fast and highly reversible redox reactions in zinc-bromine flow batteries. Journal of Power Sources 2022; 547: 232007. doi: 10.1016/j.jpowsour.2022.232007
27. Dalian Institute of Chemical Physics. Chinse Academy of Sciences. At the Dalian Institute of Chemical Physics, Chinese Academy of Sciences, our institute developed a 30KWh zinc-bromine flow battery system for the user side (Chinese). Available online: http://dicp.cas.cn/xwdt/kyjz/202201/t20220117_6344516.html (accessed on 8 May 2023).
28. Wenzhou Zinc Era Energy Co., Ltd. Available online: http://www.zinc-times.com/About.aspx?ClassID=11 (accessed on 5 September 2023).
29. Zeng YK, Zhao TS, An L, et al. A comparative study of all-vanadium and iron-chromium redox flow batteries for large-scale energy storage. Journal of Power Sources 2015; 300: 438–443. doi: 10.1016/j.jpowsour.2015.09.100
30. Wan CTC, Rodby KE, Perry ML, et al. Hydrogen evolution mitigation in iron-chromium redox flow batteries via electrochemical purification of the electrolyte. Journal of Power Sources 2023; 554: 232248. doi: 10.1016/j.jpowsour.2022.232248
31. National Development and Reform Commission. The National Development and Reform Commission of the People’s Republic of China has accelerated the development of renewable energy and added impetus to green development (Chinese). Available online: https://www.ndrc.gov.cn/fggz/hjyzy/tdftzh/202305/t20230531_1356885.html (accessed on 10 May 2023).
32. Zhang Y, Zhou C, Yang J, et al. Advances and challenges in improvement of the electrochemical performance for lead-acid batteries: A comprehensive review. Journal of Power Sources 2022; 520: 230800. doi: 10.1016/j.jpowsour.2021.230800
33. Hazza A, Pletcher D, Wills R. A novel flow battery: A lead acid battery based on an electrolyte with soluble lead (II) Part I. Preliminary studies. Physical Chemistry Chemical Physics 2004; 6(8): 1773–1778. doi: 10.1039/B401115E
34. Roberts D, Fraser EJ, Cruden A, et al. Predicting the cost of a 24 V soluble lead flow battery optimised for PV applications. Journal of Power Sources 2023; 570: 233058. doi: 10.1016/j.jpowsour.2023.233058
35. Na Z, Xu S, Yin D, et al. A cerium-lead redox flow battery system employing supporting electrolyte of methanesulfonic acid. Journal of Power Sources 2015; 295: 28–32. doi: 10.1016/j.jpowsour.2015.06.115
36. Bates A, Mukerjee S, Lee SC, et al. An analytical study of a lead-acid flow battery as an energy storage system. Journal of Power Sources 2014; 249: 207–218. doi: 10.1016/j.jpowsour.2013.10.090
37. Chang Z, Lu F, Chen R. Research on organic/inorganic redox flow batteries. In: Proceedings of the 4th National Conference on New Energy and Chemical New Materials and the National Symposium on Energy Conversion and Storage Materials; 19–21 April 2019; Dalian, China. p. 102.
38. Esser B, Dolhem F, Becuwe M, et al. A perspective on organic electrode materials and technologies for next generation batteries. Journal of Power Sources 2021; 482: 228814. doi: 10.1016/j.jpowsour.2020.228814
39. Park G, Eun S, Lee W, et al. Polybenzimidazole membrane based aqueous redox flow batteries using anthraquinone-2, 7-disulfonic acid and vanadium as redox couple. Journal of Power Sources 2023; 569: 233015. doi: 10.1016/j.jpowsour.2023.233015
40. McCormack PM, Luo H, Geise GM, et al. Conductivity, permeability, and stability properties of chemically tailored poly (phenylene oxide) membranes for Li+ conductive non-aqueous redox flow battery separators. Journal of Power Sources 2020; 460: 228107. doi: 10.1016/j.jpowsour.2020.228107
41. Pang B, Cui F, Chen W, et al. Construction of hierarchical proton sieving-conductive channels in sulfated UIO-66 grafted polybenzimidazole ion conductive membrane for vanadium redox flow battery. Journal of Power Sources 2022; 526: 231132. doi: 10.1016/j.jpowsour.2022.231132
42. Ramar A, Wang FM, Foeng R, et al. Organic redox flow battery: Are organic redox materials suited to aqueous solvents or organic solvents? Journal of Power Sources 2023; 558: 232611. doi: 10.1016/j.jpowsour.2022.232611
43. Pahlevaninezhad M, Leung P, Velasco PQ, et al. A nonaqueous organic redox flow battery using multi-electron quinone molecules. Journal of Power Sources 2021; 500: 229942. doi: 10.1016/j.jpowsour.2021.229942
44. Liu B, Tang CW, Jiang H, et al. An aqueous organic redox flow battery employing a trifunctional electroactive compound as anolyte, catholyte and supporting electrolyte. Journal of Power Sources 2020; 477: 228985. doi: 10.1016/j.jpowsour.2020.228985
45. Guiheneuf S, Godet-Bar T, Fontmorin JM, et al. A new hydroxyanthraquinone derivative with a low and reversible capacity fading process as negolyte in alkaline aqueous redox flow batteries. Journal of Power Sources 2022; 539: 231600. doi: 10.1016/j.jpowsour.2022.231600
46. Tan A, Wen Y, Huang J, et al. Multiredox tripyridine-triazine molecular cathode for lithium-organic battery. Journal of Power Sources 2023; 567: 232963. doi: 10.1016/j.jpowsour.2023.232963
47. Zhu Y, Li Y, Qian Y, et al. Anthraquinone-based anode material for aqueous redox flow batteries operating in nondemanding atmosphere. Journal of Power Sources 2021; 501: 229984. doi: 10.1016/j.jpowsour.2021.229984
48. Lei Z, Yang Q, Xu Y, et al. Boosting lithium storage in covalent organic framework via activation of 14-electron redox chemistry. Nature Communications 2018; 9(1): 576. doi: 10.1038/s41467-018-02889-7
49. Park H, Park G, Kumar S, et al. Synergistic effect of electrolyte additives on the suppression of dendrite growth in a flowless membraneless Zn-Br2 battery. Journal of Power Sources 2023; 580: 233212. doi: 10.1016/j.jpowsour.2023.233212
50. Zhang Z, Shen Y, Zhao Z, et al. Organic additives in alkaline electrolyte to improve cycling life of aqueous Zn-Ni batteries. Journal of Power Sources 2022; 542: 231815. doi: 10.1016/j.jpowsour.2022.231815
51. McArdle S, Marshall AT. Why electrode orientation and carbon felt heterogeneity can influence the performance of flow batteries. Journal of Power Sources 2023; 562: 232755. doi: 10.1016/j.jpowsour.2023.232755
52. Fu H, Bao X, He M, et al. Defect-rich graphene skin modified carbon felt as a highly enhanced electrode for vanadium redox flow batteries. Journal of Power Sources 2023; 556: 232443. doi: 10.1016/j.jpowsour.2022.232443
53. Duduta M, Ho B, Wood VC, et al. Semi-solid lithium rechargeable flow battery. Advanced Energy Materials 2011; 1(4): 511–516. doi: 10.1002/aenm.201100152
54. Borlaf M, Moreno R, Ventosa E. A new shape-conformable battery concept: The 3D printed injectable battery filled with semi-solid electrodes. Journal of Power Sources 2023; 570: 233063. doi: 10.1016/j.jpowsour.2023.233063.
55. Chen X, Zhan Y, Tang J, et al. Advances in high performance anion exchange membranes: Molecular design, preparation methods, and ion transport dynamics. Journal of Environmental Chemical Engineering 2023; 11(5): 110749. doi: 10.1016/j.jece.2023.110749
56. Cheng J, Zhou W, Zhu M, et al. Optimizing microstructure of polyelectrolyte ion exchange membrane for electrodialysis. Chemical Engineering Journal 2023; 468: 143669. doi: 10.1016/j.cej.2023.143669
57. Chen Y, Paredes-Navia SA, Romo-De-La-Cruz CO, et al. Coating internal surface of porous electrode for decreasing the ohmic resistance and shifting oxygen reduction reaction pathways in solid oxide fuel cells. Journal of Power Sources 2021; 499: 229854. doi: 10.1016/j.jpowsour.2021.229854
58. Yuan J, Pan ZZ, Jin Y, et al. Membranes in non-aqueous redox flow battery: A review. Journal of Power Sources 2021; 500: 229983. doi: 10.1016/j.jpowsour.2021.229983
59. Fang M, Qiao L, Wu M, et al. Hydrogen-bond-rich composite membrane with improved conductivity and selectivity for flow battery. Journal of Power Sources 2023; 563: 232815. doi: 10.1016/j.jpowsour.2023.232815
60. Gautam RK, Kumar A. A review of bipolar plate materials and flow field designs in the all-vanadium redox flow battery. Journal of Energy Storage 2022; 48: 104003. doi: 10.1016/j.est.2022.104003
61. Jiang F, Liao W, Ayukawa T, et al. Enhanced performance and durability of composite bipolar plate with surface modification of cactus-like carbon nanofibers. Journal of Power Sources 2021; 482: 228903. doi: 10.1016/j.jpowsour.2020.228903
62. Messaggi M, Gambaro C, Casalegno A, et al. Development of innovative flow fields in a vanadium redox flow battery: Design of channel obstructions with the aid of 3D computational fluid dynamic model and experimental validation through locally-resolved polarization curves. Journal of Power Sources 2022; 526: 231155. doi: 10.1016/j.jpowsour.2022.231155
63. Pan L, Sun J, Qi H, et al. Along-flow-path gradient flow field enabling uniform distributions of reactants for redox flow batteries. Journal of Power Sources 2023; 570: 233012. doi: 10.1016/j.jpowsour.2023.233012
64. Gundlapalli R, Bhattarai A, Ranjan R, et al. Characterization and scale-up of serpentine and interdigitated flow fields for application in commercial vanadium redox flow batteries. Journal of Power Sources 2022; 542: 231812. doi: 10.1016/j.jpowsour.2022.231812
65. Huang Z, Mu A, Wu L, et al. Vanadium redox flow batteries: Flow field design and flow rate optimization. Journal of Energy Storage 2022; 45: 103526. doi: 10.1016/j.est.2021.103526
66. Chen H, Liu Y, Zhang X, et al. Single-component slurry based lithium-ion flow battery with 3D current collectors. Journal of Power Sources 2021; 485: 229319. doi: 10.1016/j.jpowsour.2020.229319
67. Zhang Y, Park JS, Senthilkumar ST, et al. A novel rechargeable hybrid Na-seawater flow battery using bifunctional electrocatalytic carbon sponge as cathode current collector. Journal of Power Sources 2018; 400: 478–484. doi: 10.1016/j.jpowsour.2018.08.044
68. Tekaligne TM, Merso SK, Yang SC, et al. Corrosion inhibition of aluminum current collector by a newly synthesized 5-formyl-8-hydroxyquinoline for aqueous-based battery. Journal of Power Sources 2022; 550: 23214. doi: 10.1016/j.jpowsour.2022.232142