Experiments on near-field radiative heat transfer: A review
DOI:
https://doi.org/10.18686/cest.v1i1.45Keywords:
near-field radiative heat transfer; experiment; plate-to-plate; tip-to-plate; sphere-to-plateAbstract
Near-field radiative heat transfer (NFRHT) has been demonstrated to exceed the blackbody limit due to the coupling effect of evanescent waves in the near-field region, opening the door to its application in active thermal control, thermophotovoltaics, and nanoscale imaging. Although the theoretical studies on NFRHT have been investigated exhaustively, experimental measurements of NFRHT have been stagnant due to the challenges in controlling the gap distance at the nanoscale. Remarkable progress has been greatly boosted in the 21st century to overcome the nanoscale controlling and measurement of NFRHT, benefiting from the advances of micro-nanofabrication techniques and materials science. In this review, in-depth discussions on the experimental development of NFRHT are examined. According to the structure of the emitter and receiver, experimental devices are divided into three different categories: plate-to-plate structure, tip-to-plate structure, and sphere-to-plate structure. Existing experimental setups and methodology of NFRHT between metals, semiconductors, two-dimensional materials, and hyperbolic metamaterials are thoroughly explored and analyzed in detail. Finally, remarks on outstanding challenges at the nanoscale and promising advances in applications are briefly concluded in the measurements of NFRHT.
References
Wiatros-Motyka M. Global electricity review 2023. Available online: https://ember-climate.org/insights/research/global-electricity-review-2023/ (accessed on 22 September 2023).
Zhang ZM. Nano/Microscale Heat Transfer. Springer Nature; 2020.
Cuevas JC, García-Vidal FJ. Radiative heat transfer. ACS Photonics 2018; 5(10): 3896−3915. doi: 10.1021/acsphotonics.8b01031
Lucchesi C, Vaillon R, Chapuis PO. Radiative heat transfer at nanoscale: experimental trends and challenges. Nanoscale Horizons 2021; 6: 201−208. doi: 10.1039/D0NH00609B
Song B, Fiorino A, Meyhofer E, Reddy P. Near-field radiative thermal transport: From theory to experiment. AIP Advances 2015; 5(5): 053503. doi: 10.1063/1.4919048
Viloria MG, Guo Y, Merabia S, et al. Role of the Nottingham effect in heat transfer in the extreme near-field regime. Physical Review B 2023; 107(12): 125414. doi: 10.1103/PhysRevB.107.125414
Tokunaga T, Jarzembski A, Shiga T, et al. Extreme near-field heat transfer between gold surfaces. Physical Review B 2021; 104(12): 125404. doi: 10.1103/PhysRevB.104.125404
Ghashami M, Jarzembski A, Lim M, et al. Experimental exploration of near-field radiative heat transfer. Annual Review of Heat Transfer 2020; 23: 13–58. doi: 10.1615/AnnualRevHeatTransfer.2020032656
Sasihithlu K, Pendry JB, Craster RV. Van der Waals force assisted heat transfer. Zeitschrift für Naturforschung A 2017; 72(2): 181–188. doi: 10.1515/zna-2016-0361
Chiloyan V, Garg J, Esfarjani K, Chen G. Transition from near-field thermal radiation to phonon heat conduction at sub-nanometre gaps. Nature Communications 2015; 6: 6755. doi: 10.1038/ncomms7755
Biehs SA, Messina R, Venkataram PS, et al. Near-field radiative heat transfer in many-body systems. Reviews of Modern Physics 2021; 93(2): 025009. doi: 10.1103/RevModPhys.93.025009
Fiorion A, Zhu L, Thompson D, et al. Nanogap near-field thermophotovoltaics. Nature Nanotechnology 13(9): 806–811. doi: 10.1038/s41565-018-0172-5
Bhatt GR, Zhao B, Roberts S, et al. Integrated near-field thermo-photovoltaics for heat recycling. Nature Communications 2020; 11(1): 2545. doi: 10.1038/s41467-020-16197-6
Mittapally R, Lee B, Zhu L, et al. Near-field thermophotovoltaics for efficient heat to electricity conversion at high power density. Nature Communications 2021; 12(1): 4364. doi: 10.1038/s41467-021-24587-7
Yu X, Chan J, Chen C. Review of radiative cooling materials: Performance evaluation and design approaches. Nano Energy 2021; 88: 106259. doi: 10.1016/j.nanoen.2021.106259
Zhu L, Fiorino A, Thompson D, et al. Near-field photonic cooling through control of the chemical potential of photons. Nature 2019; 566(7743): 239–244. doi: 10.1038/s41586-019-0918-8
Guha B, Otey C, Poitras CB, et al. Near-field radiative cooling of nanostructures. Nano letters 2012; 12(9): 4546–4550. doi: 10.1021/nl301708e
Ren J, Li B. Preface to special topic: Phononics: Controlling thermal energy, information carried by phonons and beyond. AIP Advances 2015; 5(5): 053101. doi: 10.1063/1.4922168
Li Y, Li W, Han T, et al. Transforming heat transfer with thermal metamaterials and devices. Nature Reviews Materials 2021; 6(6): 488–507. doi: 10.48550/arXiv.2008.07964
Yin H, Zhang J, Wang X, et al. Recent progress in near-field tip enhancement: Principles and applications. Physica Status Solid-Rapid Research Letters 2022; 16: 2100456. doi: 10.1002/pssr.202100456
Seo C, Kim TT. Terahertz near-field spectroscopy for various applications. Journal of the Korean Physical Society 2022; 81(6): 549–561. doi: 10.1007/s40042-022-00404-2
Cravalho E, Domoto G, Tien C. Measurements of thermal radiation of solids at liquid-helium temperatures. In: Proceedings of 3rd Thermophysics Conference; 24–26 June 1968; Los Angeles, CA, USA. doi: 10.2514/6.1968-774
Domoto GA, Boehm RF, Tien CL. Experimental investigation of radiative transfer between metallic surfaces at cryogenic temperatures. Journal of Heat Transfer 1970; 92(3): 412–416 (1970). doi: 10.1115/1.3449677
Cravalho EG, Tien CL, Caren RP. Effect of small spacings on radiative transfer between two dielectrics. Journal of Heat Transfer 1967; 89(4): 351–358. doi: 10.1115/1.3614396
Hargreaves CM. Anomalous radiative transfer between closely-spaced bodies. Physics Letters A 30(9): 491–492. doi: 10.1016/0375-9601(69)90264-3
Polder D, Van Hove M. Theory of radiative heat transfer between closely spaced bodies. Physical Review B 1971; 4(10): 3303–3314. doi: 10.1103/PhysRevB.4.3303
Song J, Cheng Q, Zhang B, et al. Many-body near-field radiative heat transfer: methods, functionalities and applications. Reports on Progress in Physics 2021; 84(3): 036501. doi: 10.1088/1361-6633/abe52b
Lucchesi C, Vaillon R, Chapuis PO. Radiative heat transfer at the nanoscale: experimental trends and challenges. Nanoscale Horizons 2021; 6(3): 201–208. doi: 10.1039/D0NH00609B
Hu L, Narayanaswamy A, Chen X, Chen G. Near-field thermal radiation between two closely spaced glass plates exceeding Planck’s blackbody radiation law. Applied Physics Letters 2008; 92: 133106. doi: 10.1063/1.2905286
Ottens RS, Quetschke V, Wise S, et al. Near-field radiative heat transfer between macroscopic planar surfaces. Physical Review Letters 2011; 107: 014301. doi: 10.1103/PhysRevLett.107.014301
Kralik T, Hanzelka P, Zobac M, et al. Strong near-field enhancement of radiative heat transfer between metallic surfaces. Physical Review Letters 2012; 109: 224302. doi: 10.1103/PhysRevLett.109.224302
Kralik T, Hanzelka P, Musilova V, et al. Cryogenic apparatus for study of near-field heat transfer. Review of Scientific Instruments 2011; 82: 055106. doi: 10.1063/1.3585985
Feng C, Tang ZA, Yu J. A novel CMOS device capable of measuring near-field thermal radiation. Chinese Physics Letters 2012; 29(3): 038502. doi: 10.1088/0256-307X/29/3/038502
Feng C, Tang Z, Yu J, Sun C. A MEMS device capable of measuring near-field thermal radiation between membranes. Sensors 2013; 13(2): 1998–2010. doi: 10.3390/s130201998
St-Gelais R, Guha B, Zhu L, et al. Demonstration of strong near-field radiative heat transfer between integrated nanostructures. Nano Letters 2014; 14(12): 6971–6975. doi: 10.1021/nl503236k
Lim M, Lee SS, Lee BJ. Near-field thermal radiation between doped silicon plates at nanoscale gaps. Physical Review B 2015; 91: 195136. doi: 10.1103/PhysRevB.91.195136
Song B, Thompson D, Fiorino A, et al. Radiative heat conductances between dielectric and metallic parallel plates with nanoscale gaps. Nature Nanotechnology 2016; 11: 509–514. doi: 10.1038/nnano.2016.17
Bernardi MP, Milovich D, Francoeur M. Radiative heat transfer exceeding the blackbody limit between macroscale planar surfaces separated by a nanosize vacuum gap. Nature Communications 2016; 7: 12900. doi: 10.1038/ncomms12900
Ghashami M, Geng H, Kim T, et al. Precision measurement of phonon-polaritonic near-field energy transfer between macroscale planar structures under large thermal gradients. Physical Review Letters 2018; 120: 175901. doi: 10.1103/PhysRevLett.120.175901
Ito K, Miura A, Iizuka H, Toshiyoshi H. Parallel-plate submicron gap formed by micromachined low-density pillars for near-field radiative heat transfer. Applied Physics Letters 2015; 106(8): 083504. doi: 10.1063/1.4913692
Ito K, Nishikawa K, Miura A, et al. Dynamic modulation of radiative heat transfer beyond the blackbody limit. Nano Letters 2017; 17(7): 4347–4353. doi: 10.1021/acs.nanolett.7b01422
Watjen JI, Zhao B, Zhang ZM. Near-field radiative heat transfer between doped-Si parallel plates separated by a spacing down to 200 nm. Applied Physics Letters 2016; 109: 203112. doi: 10.1063/1.4967384
DeSutter J, Tang L, Francoeur M. A near-field radiative heat transfer device. Nature Nanotechnology 2019; 14: 751–755. doi: 10.1038/s41565-019-0483-1
Ying X, Sabbaghi P, Sluder N, Wang L. Super-Planckian radiative heat transfer between Macroscale surfaces with vacuum gaps down to 190 nm directly created by SU 8 posts and characterized by capacitance method. ACS Photonics 2020; 7(1): 190–196. doi: 10.1021/acsphotonics.9b01360
St-Gelais R, Zhu L, Fan S, Lipson M. Near-field radiative heat transfer between parallel structures in the deep subwavelength regime. Nature Nanotechnology 2016; 11: 515–519. doi: 10.1038/nnano.2016.20
Fiorino A, Thompson D, Zhu L, et al. Giant enhancement in radiative heat transfer in Sub-30 nm gaps of plane parallel surfaces. Nano Letters 2018; 18(6): 3711–3715. doi: 10.1021/acs.nanolett.8b00846
Salihoglu H, Nam W, Traverso L, et al. Near-Field thermal radiation between two plates with sub-10 nm vacuum separation. Nano letters 2020; 20(8): 6091–6096. doi: 10.1021/acs.nanolett.0c02137
Fiorino A, Thompson D, Zhu L, et al. A thermal diode based on nanoscale thermal radiation. ACS Nano 2018; 12(6): 5774–5779. doi: 10.1021/acsnano.8b01645
Thompson D, Zhu L, Meyhofer E, et al. Nanoscale radiative thermal switching via multi-body effects. Nature Nanotechnology 2020; 15: 99–104. doi: 10.1038/s41565-019-0595-7
Messina R, Ben-Abdallah P. Graphene-based photovoltaic cells for near-field thermal energy conversion. Scientific Reports 2013; 3: 1383. doi: 10.1038/srep01383
Svetovoy VB, Palasantzas G. Graphene-on-silicon near-field thermophotovoltaic cell. Physical Review Applied 2014; 2: 034006. doi: 10.1103/PhysRevApplied.2.034006
Ghosh S, Calizo I, Teweldebrhan D, et al. Extremely high thermal conductivity of graphene: Prospects for thermal management applications in nanoelectronic circuits. Applied Physics Letters 2008; 92(15): 151911. doi: 10.1063/1.2907977
Yang J, Du W, Su Y, et al. Observing of the super-Planckian near-field thermal radiation between graphene sheets. Nature Communications 2018; 9: 4033. doi: 10.1038/s41467-018-06163-8
Shi K, Sun Y, Chen Z, et al. Colossal enhancement of near-field thermal radiation across hundreds of nanometers between millimeter-scale plates through surface plasmon and phonon polaritons coupling. Nano Letters 2019; 19(11): 8082–8088. doi: 10.1021/acs.nanolett.9b03269
Shi K, Chen Z, Xu X, et al. Optimized colossal near-field thermal radiation enabled by manipulating coupled plasmon polariton geometry. Advanced Materials 2021; 33(52): 2106097. doi: 10.1002/adma.202106097
Shi K, Chen Z, Xing X, et al. Near-Field Radiative Heat Transfer Modulation with an Ultrahigh Dynamic Range through Mode Mismatching. Nano Letters 2022; 22(19): 7753–7760. doi: 10.1021/acs.nanolett.2c01286
Lu L, Zhang B, Ou H, et al. Enhanced near-field radiative heat transfer between graphene/hBN systems. Small 2022; 18(19): 2108032. doi: 10.1002/smll.202108032
Thomas NH, Sherrott MC, Broulliet J, et al. Electronic modulation of near-field radiative transfer in graphene field effect heterostructures. Nano Letters 2019; 19(6): 3898–3904. doi: 10.1021/acs.nanolett.9b01086
Shi J, Liu B, Li P, et al. Near-field energy extraction with hyperbolic metamaterials. Nano Letters 2015; 15(2): 1217–1221. doi: 10.1021/nl504332t
Du W, Yang J, Zhang S, et al. Super-Planckian near-field heat transfer between hyperbolic metamaterials. Nano Energy 2020; 78: 105264. doi: 10.1016/j.nanoen.2020.105264
Lim M, Song J, Lee SS, Lee BJ. Tailoring near-field thermal radiation between metallo-dielectric multilayers using coupled surface plasmon polaritons. Nature Communications 2018; 9: 4302. doi: 10.1038/s41467-018-06795-w
Sabbaghi P, Long L, Ying X, et al. Super-Planckian radiative heat transfer between macroscale metallic surfaces due to near-field and thin-film effects. Journal of Applied Physics 2020; 128(2): 025305. doi: 10.1063/5.0008259
Lim M, Son J. Surface-plasmon-enhanced near-field radiative heat transfer between planar surfaces with a thin-film plasmonic coupler. Physics Review Applied 2020; 14(1): 014070. doi: 10.1103/PhysRevApplied.14.014070
Tang L, DeSutter J, Francoeur M. Near-field radiative heat transfer between dissimilar materials mediated by coupled surface phonon- and plasmon-polaritons. ACS Photonics 2020; 7(5): 1304–1311. doi: 10.1021/acsphotonics.0c00404
Iqbal N, Zhang S, Wang S, et al. Near-field radiative heat transfer between hybrid polaritonic structures. arXiv 2022; arXiv:2202.13712. doi: 10.48550/arXiv.2202.13712
Williams CC, Wickramasinghe HK. Scanning thermal profiler. Microelectronic Engineering 1986; 5(1–4): 509–513. doi: 10.1016/0167-9317(86)90084-5
Dransfeld K, Xu J. The heat transfer between a heated tip and a substrate: Fast thermal microscopy. Journal of Microscopy 1988; 152(1): 35–42. doi: 10.1111/j.1365-2818.1988.tb01359.x
Müller-Hirsch W, Kraft A, Hirsch MT, et al. Heat transfer in ultrahigh vacuum scanning thermal microscopy. Journal of Vacuum Science & Technology A 1999; 17(4): 1205–1210. doi: 10.1116/1.581796
Kittel A, Müller-Hirsch W, Parisi J, et al. Near-field heat transfer in a scanning thermal microscope. Physical Review Letters 2005; 95(22): 224301. doi: 10.1103/PhysRevLett.95.224301
Kittel A, Wischnath UF, Welker J, et al. Near-field thermal imaging of nanostructured surfaces. Applied Physics Letters 2008; 93(19): 193109. doi: 10.1063/1.3025140
Wischnath UF, Welker J, Munzel M, Kittel A. The near-field scanning thermal microscope. Review of Scientific Instruments 2008; 79(7): 073708. doi: 10.1063/1.2955764
Worbes L, Hellmann D, Kittel A. Enhanced near-field heat flow of a monolayer dielectric island. Physical Review Letters 2013; 110(13): 134302. doi: 10.1103/PhysRevLett.110.134302
Kim K, Song B, Fernández-Hurtado V, Lee W. Radiative heat transfer in the extreme near field. Nature 2015; 528: 387–391. doi: 10.1038/nature16070
Kloppstech K, Konne N, Biehs S, et al. Giant heat transfer in the crossover regime between conduction and radiation. Nature Communications 2017; 8: 14475. doi: 10.1038/ncomms14475
Cui L, Jeong W, Fernández-Hurtado V, et al. Study of radiative heat transfer in Ångström- and nanometre-sized gaps. Nature Communications 2017; 8(1): 14479. doi: 10.1038/ncomms14479
Narayanaswamy A, Shen S, Chen G. Near-field radiative heat transfer between a sphere and a substrate. Physical Review B 2008; 79(11): 115303. doi: 10.1103/PhysRevB.78.115303
Shen S, Narayanaswamy A, Chen G. Surface phonon polaritons mediated energy transfer between nanoscale gaps. Nano Letters 2009; 9(8): 2909–2913. doi: 10.1021/nl901208v
Shen S, Mavrokefalos A, Sambegoro P, Chen G. Nanoscale thermal radiation between two gold surfaces. Applied Physics Letters 2012; 100: 233114. doi: 10.1063/1.4723713
Shi J, Li P, Liu B, Shen S. Tuning near field radiation by doped silicon. Applied Physics Letters 2013; 102(18): 183114. doi: 10.1063/1.4804631
Rousseau E, Siria A, Jourdan G, et al. Radiative heat transfer at the nanoscale. Nature Photonics 2009; 3(9): 514–517. doi: 10.1038/nphoton.2009.144
Van Zwol PJ, Ranno L, Chevrier J. Tuning near field radiative heat flux through surface excitations with a metal insulator transition. Physical Review Letters 2012; 108(23): 234301. doi: 10.1103/PhysRevLett.108.234301
Van Zwol PJ, Thiele S, Berger C, et al. Nanoscale radiative heat flow due to surface plasmons in graphene and doped Silicon. Physical Review Letters 2012; 109(26): 264301. doi: 10.1103/PhysRevLett.109.264301
Cahill DG, Braun PV, Chen G, et al. Nanoscale thermal transport. II. 2003–2012. Applied Physics Reviews 2014; 1(1): 011305. doi: 10.1063/1.4832615
Song B, Ganjeh Y, Sadat S, et al. Enhancement of near-field radiative heat transfer using polar dielectric thin films. Nature Nanotechnology 2015; 10(3): 253–258. doi: 10.1038/nnano.2015.6
Biehs SA, Ben-Abdallah P, Rosa FSS, et al. Nanoscale heat flux between nanoporous materials. Optics Express 2011; 19(S5): A1088–A1103. doi: 10.1364/OE.19.0A1088
Wu X, Fu C, Zhang Z. Near-field radiative heat transfer between two α-MoO3 biaxial crystals. Journal of Heat Transfer 2020; 142: 072802. doi: 10.1115/1.4046968
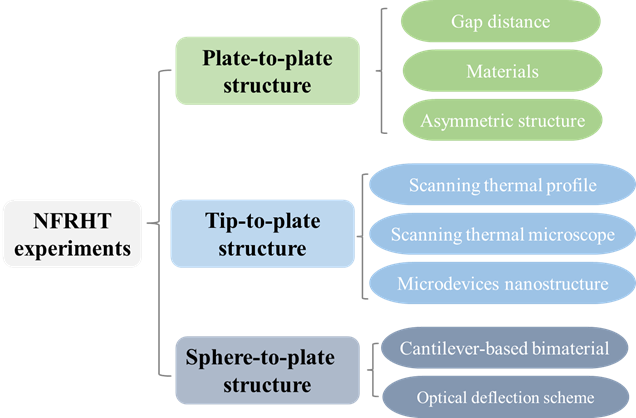
Downloads
Published
How to Cite
Issue
Section
License
Copyright (c) 2023 Jihong Zhang, Kezhang Shi, Lu Lu, Dudong Feng, Haotuo Liu, Xiaohu Wu

This work is licensed under a Creative Commons Attribution-NonCommercial 4.0 International License.