Recent progress in carbon dioxide capture technologies: A review
DOI:
https://doi.org/10.18686/cest.v1i1.32Keywords:
post-combustion technology; amine capture processAbstract
The continuous increase in anthropogenic CO2 emissions is widely acknowledged as one of the main reasons for global climate change. To address this issue, significant advancements have been made in developing CO2 capture and utilization technologies that offer new solutions for mitigating carbon emissions and promoting a carbon economy. In this review, we summarize the recent research progress in CO2 capture and separation technologies, including pre-combustion, post-combustion, oxy-fuel combustion, chemical looping combustion and calcium looping combustion. Among these technologies, post-combustion is seen as one of the most promising options for reducing CO2 emissions from existing power plants, as it can be easily integrated into existing facilities without requiring major modifications. Therefore, the second section of this article focuses on the various post-combustion processes and technologies, such as physical absorption, amine scrubbing, dual-alkali absorption, chilled ammonia, membrane separation, and solid adsorption, with a particular emphasis on most recent research reports. As amine-based chemical absorption is the most leading post-combustion CO2 capture technique, the third section summarizes the recent development in amine-based absorption technology by covering conventional and emerging types of absorbents such as single amine, blended amine, biphasic amine, and non-aqueous amine processes. The different liquid absorption-based process is compared in terms of regeneration energy consumption, CO2 intake capacity, and optimal operating conditions, and the comparison data is summarized in tables. A critical literature review and comparison of various techniques show that non-aqueous amine absorbents can be promising alternatives to the conventional monoethanolamine (MEA) process. The goal of this review is to provide strategies and perspectives for accelerating the further study and development of CCS technologies.
References
Nguyen DN. Carbon dioxide geological sequestration: Technical and economic reviews. In: Proceedings of the SPE/EPA/DOW Exploration and Production Environmrntal Conference; March 2003; United States. pp. 249–254.
Marchetti C. On geoengineering and the CO2 problem. Climatic Change 1977; 1(1): 59–68. doi: 10.1007/BF00162777
Metz B, Davidson O, De Coninck HC, et al. The IPCC special report on carbon dioxide capture and storage. In: Proceedings of the IPCC WGIII 8th Session; 22–24 September 2005; Montreal, Canada.
Pardemann R, Meyer B. Pre-combustion carbon capture. In: Yan J (editor). Handbook of Clean Energy Systems. Wiley; 2015. doi: 10.1002/9781118991978.hces061
Bui M, Adjiman CS, Bardow A, et al. Carbon capture and storage (CCS): The way forward. Energy & Environmental Science 2018; 11(5): 1062–1176. doi: 10.1039/C7EE02342A
Sgouridis S, Carbajales-Dale M, Csala D, et al. Comparative net energy analysis of renewable electricity and carbon capture and storage. Naure Energy 2019; 4(6): 456–465. doi: 10.1038/s41560-019-0365-7
Romanak K, Fridahl M, Dixon T. Attitudes on carbon capture and storage (CCS) as a mitigation technology within the UNFCCC. Energies 2021; 14(3): 629. doi: 10.3390/EN14030629
Al-Mamoori A, Krishnamurthy A, Rownaghi AA, Rezaei F. Carbon capture and utilization update. Energy Technology 2017; 5(6): 834–849. doi: 10.1002/ente.201600747
Boot-Handford ME, Abanades JC, Anthony EJ, et al. Carbon capture and storage update. Energy & Environmental Science 2014; 7(1): 130–189. doi: 10.1039/C3EE42350F
Leung DYC, Caramanna G, Maroto-Valer MM. An overview of current status of carbon dioxide capture and storage technologies. Renewable and Sustainable Energy Reviews 2014; 39: 426–443. doi: 10.1016/j.rser.2014.07.093
Markewitz P, Kuckshinrichs W, Leitner W, et al. Worldwide innovations in the development of carbon capture technologies and the utilization of CO2. Energy & Environmental Science 2012; 5(6): 7281–7305. doi: 10.1039/C2EE03403D
Naims H. Economics of carbon dioxide capture and utilization—A supply and demand perspective. Environmental Science and Pollution Research 2016; 23: 22226–22241. doi: 10.1007/S11356-016-6810-2
Ho HJ, Iizuka A, Shibata E. Carbon capture and utilization technology without carbon dioxide purification and pressurization: A review on its necessity and available technologies. Industrial & Engineering Chemistry Research 2019; 58(21): 8941–8954. doi: 10.1021/ACS.IECR.9B01213
Duan X, Xu J, Wei Z, et al. Metal‐free carbon materials for CO2 electrochemical reduction. Advanced Materials 2017; 29(41): 1701784. doi: 10.1002/adma.201701784
Nam DH, De Luna P, Rosas-Hernández A, et al. Molecular enhancement of heterogeneous CO2 reduction. Nature materials 2020; 19(3): 266–276. doi: 10.1038/s41563-020-0610-2
Chen C, Kotyk JFK, Sheehan SW. Progress toward commercial application of electrochemical carbon dioxide reduction. Chem 2018; 4(11): 2571–2586. doi: 10.1016/J.CHEMPR.2018.08.019
Koytsoumpa EI, Bergins C, Kakaras E. The CO2 economy: Review of CO2 capture and reuse technologies. Journal of Supercritical Fluids 2018; 132: 3–16. doi: 10.1016/j.supflu.2017.07.029
Aresta M, Dibenedetto A, Angelini A. The changing paradigm in CO2 utilization. Journal of CO2 Utilization 2013; 3: 65–73. doi: 10.1016/J.JCOU.2013.08.001
Mac Dowell N, Fennell PS, Shah N, et al. The role of CO2 capture and utilization in mitigating climate change. Nature Climate Change 2017; 7(4): 243–249. doi: 10.1038/nclimate3231
Lockwood T. A compararitive review of next-generation carbon capture technologies for coal-fired power plant. Energy procedia 2017; 114: 2658–2670. doi: 10.1016/j.egypro.2017.03.1850
Sifat NS, Haseli Y. A critical review of CO2 capture technologies and prospects for clean power generation. Energies 2019; 12(21): 4143. doi: 10.3390/en12214143
Chao C, Deng Y, Dewil R, et al. Post-combustion carbon capture. Renewable and Sustainable Energy Reviews 2021; 138: 110490. doi: 10.1016/j.rser.2020.110490
Song QW, Zhou ZH, He LN. Efficient, selective and sustainable catalysis of carbon dioxide. Green Chemistry 2017; 19(16): 3707–3728. doi: 10.1039/C7GC00199A
Zhu X, Xie W, Wu J, et al. Recent advances in direct air capture by adsorption. Chemical Society Reviews 2022; 51: 6574–6651. doi: 10.1039/D1CS00970B
Fasihi M, Efimova O, Breyer C. Techno-economic assessment of CO2 direct air capture plants. Journal of Cleaner Production 2019; 224: 957–980. doi: 10.1016/j.jclepro.2019.03.086
Shi X, Xiao H, Azarabadi H, et al. Sorbents for the direct capture of CO2 from ambient air. Angewandte Chemie International Edition 2020; 59(18): 6984–7006. doi: 10.1002/anie.201906756
McQueen N, Gomes KV, McCormick C, et al. A review of direct air capture (DAC): Scaling up commercial technologies and innovating for the future. Progress in Energy 2021; 3(3): 032001.
Feron PHM, Hendriks CA. CO2 capture process principles and costs (French). Oil & Gas Science and Technology 2005; 60(3): 451–459. doi: 10.2516/ogst:2005027
Irons R, Sekkapan G, Panesar R, et al. CO2 capture ready plants. IEA Greenhouse Gas Programme 2007.
Global CCS Institute. CO2 capture technologies: Precombustion with CO2 capture. Available online: https://www.globalccsinstitute.com/archive/hub/publications/29756/co2-capture-technologies-pre-combustion-capture.pdf (accessed on 8 September 2023).
Olabi AG, Obaideen K, Elsaid K, et al. Assessment of the pre-combustion carbon capture contribution into sustainable development goals SDGs using novel indicators. Renewable and Sustainable Energy Reviews 2022; 153: 111710. doi: 10.1016/j.rser.2021.111710
Park SH, Lee SJ, Lee JW, et al. The quantitative evaluation of two-stage pre-combustion CO2 capture processes using the physical solvents with various design parameters. Energy 2015; 81: 47–55. doi: 10.1016/j.energy.2014.10.055
Jiang G, Huang Q, Kenarsari SD, et al. A new mesoporous amine-TiO2 based pre-combustion CO2 capture technology. Applied Energy 2015; 147: 214–223. doi: 10.1016/j.apenergy.2015.01.081
Dai Z, Deng L. Membrane absorption using ionic liquid for pre-combustion CO2 capture at elevated pressure and temperature. International Journal of Greenhouse Gas Control 2016; 54: 59–69. doi: 10.1016/j.ijggc.2016.09.001
Yang M, Jing W, Zhao J, et al. Promotion of hydrate-based CO2 capture from flue gas by additive mixtures (THF (tetrahydrofuran)+TBAB (tetra-n-butyl ammonium bromide)). Energy 2016; 106: 546–553. doi: 10.1016/j.energy.2016.03.092
Usman M, Hillestad M, Deng L. Assessment of a membrane contactor process for pre-combustion CO2 capture by modelling and integrated process simulation. International Journal of Greenhouse Gas Control 2018; 71: 95–103. doi: 10.1016/j.ijggc.2018.02.012
Zhai H, Rubin ES. Systems analysis of physical absorption of CO2 in ionic liquids for pre-combustion carbon capture. Environmental Science & Technology 2018; 52(8): 4996–5004. doi: 10.1021/acs.est.8b00411
D’Alessandro DM, Smit B, Long JR. Carbon dioxide capture: Prospects for new materials. Angewandte Chemie International Edition 2010; 49(35): 6058–6082. doi: 10.1002/anie.201000431
Plaza MG, García S, Rubiera F, et al. Post-combustion CO2 capture with a commercial activated carbon: Comparison of different regeneration strategies. Chemical Engineering Journal 2010; 163(1–2): 41–47. doi: 10.1016/j.cej.2010.07.030
Salvi BL, Jindal S. Recent developments and challenges ahead in carbon capture and sequestration technologies. SN Applied Sciences 2019; 1: 1–20. doi: 10.1007/s42452-019-0909-2
Idem R, Wilson M, Tontiwachwuthikul P, et al. Pilot plant studies of the CO2 capture performance of aqueous MEA and mixed MEA/MDEA solvents at the University of Regina CO2 capture technology development plant and the boundary dam CO2 capture demonstration plant. Industrial & Engineering Chemistry Research 2006; 45(8): 2414–2420. doi: 10.1021/ie050569e
Ahn H, Luberti M, Liu Z, et al. Process configuration studies of the amine capture process for coal-fired power plants. International Journal of Greenhouse Gas Control 2013; 16: 29–40. doi: 10.1016/j.ijggc.2013.03.002
Bougie F, Fan X. Microwave regeneration of monoethanolamine aqueous solutions used for CO2 capture. International Journal of Greenhouse Gas Control 2018; 79: 165–172. doi: 10.1016/j.ijggc.2018.10.008
Zhang Z. Comparisons of various absorbent effects on carbon dioxide capture in membrane gas absorption (MGA) process. Journal of Natural Gas Science and Engineering 2016; 31: 589–595. doi: 10.1016/j.jngse.2016.03.052
El Hadri N, Quang DV, Goetheer ELV, et al. Aqueous amine solution characterization for post-combustion CO2 capture process. Applied Energy 2017; 185: 1433–1449. doi: 10.1016/j.apenergy.2016.03.043
Thompson JG, Combs M, Abad K, et al. Pilot testing of a heat integrated 0.7 MWe CO2 capture system with two-stage air-stripping: Emission. International Journal of Greenhouse Gas Control 2017; 64: 267–275. doi: 10.1016/j.ijggc.2017.08.003
Zhang Z, Chen F, Rezakazemi M, et al. Modeling of a CO2-piperazine-membrane absorption system. Chemical Engineering Research and Design 2018; 131: 375–384. doi: 10.1016/j.cherd.2017.11.024
Bougie F, Pokras D, Fan X. Novel non-aqueous MEA solutions for CO2 capture. International Journal of Greenhouse Gas Control 2019; 86: 34–42. doi: 10.1016/j.ijggc.2019.04.013
Toftegaard MB, Brix J, Jensen PA, et al. Oxy-fuel combustion of solid fuels. Progress in Energy and Combustion Science 2010; 36(5): 581–625. doi: 10.1016/j.pecs.2010.02.001
Nemitallah MA, Habib MA, Badr HM, et al. Oxy-fuel combustion technology: current status, applications, and trends. International Journal of Energy Research 2017; 41(12): 1670–1708. doi: 10.1002/er.3722
Vellini M, Gambini M. CO2 capture in advanced power plants fed by coal and equipped with OTM. International Journal of Greenhouse Gas Control 2015; 36: 144–152. doi: 10.1016/j.ijggc.2015.01.022
Falkenstein-Smith R, Zeng P, Ahn J. Investigation of oxygen transport membrane reactors for oxy-fuel combustion and carbon capture purposes. Proceedings of the Combustion Institute 2017; 36(3): 3969–3976. doi: 10.1016/j.proci.2016.09.005
Ferrari N, Mancuso L, Davison J, et al. Oxy-turbine for Power Plant with CO2 capture. Energy Procedia 2017; 114: 471–480. doi: 10.1016/j.egypro.2017.03.1189
Laumb JD, Holmes MJ, Stanislowski JJ, et al. Supercritical CO2 cycles for power production. Energy Procedia 2017; 114: 573–580. doi: 10.1016/j.egypro.2017.03.1199
Choi BS, Kim MJ, Ahn JH, et al. Influence of a recuperator on the performance of the semi-closed oxy-fuel combustion combined cycle. Applied Thermal Engineering 2017; 124: 1301–1311. doi: 10.1016/j.applthermaleng.2017.06.055
Chen S. Fundamentals of oxy-fuel combustion. In: Zheng C, Liu Z (editors). Oxy-fuel Combustion: Fundamentals, Theory and Practice. Elsevier BV; 2018. pp. 13–30.
Benson SM, Orr FM. Carbon dioxide capture and storage. MRS Bulletin 2008; 33(4): 303–305.
Adanez J, Abad A, Garcia-Labiano F, et al. Progress in chemical-looping combustion and reforming technologies. Progress in Energy and Combustion Science 2012; 38(2): 215–282. doi: 10.1016/j.pecs.2011.09.001
Jin H, Ishida M. A new type of coal gas fueled chemical-looping combustion. Fuel 2004; 83(17–18): 2411–2417. doi: 10.1016/j.fuel.2004.06.033
Li J, Zhang H, Gao Z, et al. CO2 capture with chemical looping combustion of gaseous fuels: An overview. Energy & Fuels 2017; 31(4): 3475–3524. doi: 10.1021/acs.energyfuels.6b03204
Lyngfelt A. Chemical looping combustion: status and development challenges. Energy & Fuels 2020; 34(8): 9077–9093. doi: 10.1021/acs.energyfuels.0c01454
Olajire AA. CO2 capture and separation technologies for end-of-pipe applications—A review. Energy 2010; 35(6): 2610–2628. doi: 10.1016/j.energy.2010.02.030
Erlach B, Schmidt M, Tsatsaronis G. Comparison of carbon capture IGCC with pre-combustion decarbonisation and with chemical-looping combustion. Energy 2011; 36(6): 3804–3815. doi: 10.1016/j.energy.2010.08.038
Johansson E, Mattisson T, Lyngfelt A, et al. Combustion of syngas and natural gas in a 300 W chemical-looping combustor. Chemical Engineering Research and Design 2006; 84(9): 819–827. doi: 10.1205/cherd05024
Lyngfelt A, Leckner B. A 1000 MWth boiler for chemical-looping combustion of solid fuels—Discussion of design and costs. Applied Energy 2015; 157: 475–487. doi: 10.1016/j.apenergy.2015.04.057
Abad A, Adánez J, Gayán P, et al. Conceptual design of a 100 MWth CLC unit for solid fuel combustion. Applied Energy 2015; 157: 462–474. doi: 10.1016/j.apenergy.2015.04.043
Mendiara T, Pérez-Astray A, Izquierdo M T, et al. Chemical looping combustion of different types of biomass in a 0.5 kWth unit. Fuel 2018; 211: 868–875. doi: 10.1016/j.fuel.2017.09.113
Ohlemüller P, Busch JP, Reitz M, et al. Chemical-looping combustion of hard coal: Autothermal operation of a 1 MWth pilot plant. Journal of Energy Resources Technology 2016; 138(4): 042203. doi: 10.1115/1.4032357
Ohlemüller P, Reitz M, Ströhle J, et al. Investigation of chemical looping combustion of natural gas at 1 MWth scale. Proceedings of the Combustion Institute 2019; 37(4): 4353–4360. doi: 10.1016/j.proci.2018.07.035
Abad A, Gayán P, Pérez-Vega R, et al. Evaluation of different strategies to improve the efficiency of coal conversion in a 50 kWth Chemical Looping combustion unit. Fuel 2020; 271: 117514. doi: 10.1016/j.fuel.2020.117514
Matzen M, Pinkerton J, Wang X, et al. Use of natural ores as oxygen carriers in chemical looping combustion: A review. International Journal of Greenhouse Gas Control 2017; 65: 1–14. doi: 10.1016/j.ijggc.2017.08.008
Adánez-Rubio I, Pérez-Astray A, Mendiara T, et al. Chemical looping combustion of biomass: CLOU experiments with a Cu-Mn mixed oxide. Fuel Processing Technology 2018; 172: 179–186. doi: 10.1016/j.fuproc.2017.12.010
Liu F, Liu J, Li Y, et al. Studies on the synergistically improved reactivity of spinel NiFe2O4 oxygen carrier for chemical-looping combustion. Energy 2022; 239: 122100. doi: 10.1016/j.energy.2021.122100
Fennell P. Calcium and chemical looping technology: An introduction. In: Fennell P, Anthony B (editors). Calcium and Chemical Looping Technology for Power Generation and Carbon Dioxide (CO2) Capture. Elsevier Science; 2015. pp. 3–14.
Blamey J, Anthony EJ, Wang J, et al. The calcium looping cycle for large-scale CO2 capture. Progress in Energy and Combustion Science 2010; 36(2): 260–279. doi: 10.1016/j.pecs.2009.10.001
Bui M, Adjiman CS, Bardow A, et al. Carbon capture and storage (CCS): The way forward. Energy & Environmental Science 2018; 11(5): 1062–1176. doi: 10.1039/C7EE02342A
Silaban A, Harrison DP. High temperature capture of carbon dioxide: Characteristics of the reversible reaction between CaO (s) and CO2 (g). Chemical Engineering Communications 1995; 137(1): 177–190. doi: 10.1080/00986449508936375
Adams TA, Hoseinzade L, Madabhushi PB, et al. Comparison of CO2 capture approaches for fossil-based power generation: Review and meta-study. Processes 2017; 5(3): 44. doi: 10.3390/pr5030044
Hanak DP, Manovic V. Calcium looping combustion for high-efficiency low-emission power generation. Journal of Cleaner Production 2017; 161: 245–255. doi: 10.1016/j.jclepro.2017.05.080
Barker R. The reversibility of the reaction CaCO3 ⇄ CaO + CO2. Journal of Applied Chemistry and Biotechnology 1973; 23(10): 733–742. doi: 10.1002/jctb.5020231005
Erans M, Manovic V, Anthony EJ. Calcium looping sorbents for CO2 capture. Applied Energy 2016; 180: 722–742. doi: 10.1016/j.apenergy.2016.07.074
Valverde JM, Sanchez-Jimenez PE, Pérez-Maqueda LA. Ca-looping for postcombustion CO2 capture: A comparative analysis on the performances of dolomite and limestone. Applied Energy 2015; 138: 202–215. doi: 10.1016/j.apenergy.2014.10.087
Shan SY, Ma AH, Hu YC, et al. Development of sintering-resistant CaO-based sorbent derived from eggshells and bauxite tailings for cyclic CO2 capture. Environmental Pollution 2016; 208: 546–552. doi: 10.1016/j.envpol.2015.10.028
González B, Blamey J, Al-Jeboori MJ, et al. Additive effects of steam addition and HBr doping for CaO-based sorbents for CO2 capture. Chemical Engineering and Processing: Process Intensification 2016; 103: 21–26. doi: 10.1016/j.cep.2015.09.019
Ma J, Mei D, Peng W, et al. On the high performance of a core-shell structured CaO-CuO/MgO@ Al2O3 material in calcium looping integrated with chemical looping combustion (CaL-CLC). Chemical Engineering Journal 2019; 368: 504–512. doi: 10.1016/j.cej.2019.02.188
Duhoux B, Mehrani P, Lu DY, et al. Combined calcium looping and chemical looping combustion for post‐combustion carbon dioxide capture: Process simulation and sensitivity analysis. Energy Technology 2016; 4(10): 1158–1170. doi: 10.1002/ente.201600024
Krzywanski J, Czakiert T, Shimizu T, et al. NOx emissions from regenerator of calcium looping process. Energy & Fuels 2018; 32(5): 6355–6362. doi: 10.1021/acs.energyfuels.8b00944
Michalski S, Hanak DP, Manovic V. Advanced power cycles for coal-fired power plants based on calcium looping combustion: A techno-economic feasibility assessment. Applied Energy 2020; 269: 114954. doi: 10.1016/j.apenergy.2020.114954
Hanak DP, Michalski S, Manovic V. Supercritical CO2 cycle for coal-fired power plant based on calcium looping combustion. Thermal Science and Engineering Progress 2020; 20: 100723. doi: 10.1016/j.tsep.2020.100723
Wang S, Wang X. Imidazolium ionic liquids, imidazolylidene heterocyclic carbenes, and zeolitic imidazolate frameworks for CO2 capture and photochemical reduction. Angewandte Chemie International Edition 2016; 55(7): 2308–2320. doi: 10.1002/anie.201507145
Sun S, Sun H, Williams PT, Wu C. Recent advances in integrated CO2 capture and utilization: A review. Sustainable Energy & Fuels 2021; 5(18): 4546–4559. doi: 10.1039/D1SE00797A.
Aziz MAA, Jalil AA, Triwahyono S, et al. CO2 methanation over heterogeneous catalysts: Recent progress and future prospects. Green Chemistry 2015; 17(5): 2647–2663. doi: 10.1039/C5GC00119F
Bradford MCJ, Vannice MA. The role of metal–support interactions in CO2 reforming of CH4. Catalysis Today 1999; 50(1): 87–96. doi: 10.1016/S0920-5861(98)00465-9
Stagg-Williams SM, Noronha FB, Fendley G, et al. CO2 reforming of CH4 over Pt/ZrO2 catalysts promoted with La and Ce oxides. Journal of Catalysis 2000; 194(2): 240–249. doi: 10.1006/jcat.2000.2939
Usman M, Daud WMAW, Abbas HF. Dry reforming of methane: Influence of process parameters—A review. Renewable and Sustainable Energy Reviews 2015; 45: 710–744. doi: 10.1016/j.rser.2015.02.026
Aramouni NAK, Touma JG, Tarboush BA, et al. Catalyst design for dry reforming of methane: Analysis review. Renewable and Sustainable Energy Reviews 2018; 82: 2570–2585. doi: 10.1016/j.rser.2017.09.076
Abdullah B, Abd Ghani NA, Vo DVN. Recent advances in dry reforming of methane over Ni-based catalysts. Journal of Cleaner Production 2017; 162: 170–185. doi: 10.1016/j.jclepro.2017.05.176
Tian S, Yan F, Zhang Z, et al. Calcium-looping reforming of methane realizes in situ CO2 utilization with improved energy efficiency. Science Advances 2019; 5(4): eaav5077. doi: 10.1126/sciadv.aav5077
Hu Y, Xu Q, Sheng Y, et al. Catalytic synthesis of CO by combining CO2 capture and hydrogenation over three-dimensional Ni/CaO networks. Energy & Fuels 2023; 37(11): 7871–7880. doi: 10.1021/acs.energyfuels.2c04368
Zhu M, Tian P, Cao X, et al. Vacancy engineering of the nickel-based catalysts for enhanced CO2 methanation. Applied Catalysis B: Environmental 2021; 282: 119561. doi: 10.1016/j.apcatb.2020.119561
Zhao Y, Jin B, Liang Z. Synergistic enhanced Ca–Fe chemical looping reforming process for integrated CO2 capture and conversion. Industrial & Engineering Chemistry Research 2019; 59(3): 1298–1307. doi: 10.1021/acs.iecr.9b05783
Sun S, Lv Z, Qiao Y, et al. Integrated CO2 capture and utilization with CaO-alone for high purity syngas production. Carbon Capture Science & Technology 2021; 1: 100001. doi: 10.1016/j.ccst.2021.100001
Oschatz M, Antonietti M. A search for selectivity to enable CO2 capture with porous adsorbents. Energy & Environmental Science 2018; 11(1): 57–70. doi: 10.1039/C7EE02110K
Sun X, Zhu L, Wang P, et al. CO2 removal from natural gas by moisture swing adsorption. Chemical Engineering Research and Design 2021; 176: 162–168. doi: 10.1016/j.cherd.2021.09.033
Voskian S, Hatton TA. Faradaic electro-swing reactive adsorption for CO2 capture. Energy & Environmental Science 2019; 12(12): 3530–3547. doi: 10.1039/C9EE02412C
McQueen N, Kelemen P, Dipple G, et al. Ambient weathering of magnesium oxide for CO2 removal from air. Nature Communications 2020; 11(1): 3299. doi: 10.1038/s41467-020-16510-3
Brethomé FM, Williams NJ, Seipp CA, et al. Direct air capture of CO2 via aqueous-phase absorption and crystalline-phase release using concentrated solar power. Nature Energy 2018; 3(7): 553–559. doi: 10.1038/s41560-018-0150-z
Holmes G, Keith DW. An air–liquid contactor for large-scale capture of CO2 from air. Philosophical Transactions of the Royal Society A: Mathematical, Physical and Engineering Sciences 2012; 370(1974): 4380–4403. doi: 10.1098/rsta.2012.0137
Darunte LA, Oetomo AD, Walton KS, et al. Direct air capture of CO2 using amine functionalized MIL-101 (Cr). ACS Sustainable Chemistry & Engineering 2016; 4(10): 5761–5768. doi: 10.1021/acssuschemeng.6b01692
Erans M, Sanz-Pérez ES, Hanak DP, et al. Direct air capture: Process technology, techno-economic and socio-political challenges. Energy & Environmental Science 2022; 15(4): 1360–1405. doi: 10.1039/D1EE03523A
Boone P, He Y, Lieber AR, et al. Designing optimal core–shell MOFs for direct air capture. Nanoscale 2022; 14(43): 16085–16096. doi: 10.1039/D2NR03177A
McQueen N, Psarras P, Pilorgé H, et al. Cost analysis of direct air capture and sequestration coupled to low-carbon thermal energy in the United States. Environmental Science & Technology 2020; 54(12): 7542–7551. doi: 10.1021/acs.est.0c00476
Wu X, Krishnamoorti R, Bollini P. Technological options for direct air capture: A comparative process engineering review. Annual Review of Chemical and Biomolecular Engineering 2022; 13: 279–300. doi: 10.1146/annurev-chembioeng-102121-065047
Yousef AM, El-Maghlany WM, Eldrainy YA, et al. New approach for biogas purification using cryogenic separation and distillation process for CO2 capture. Energy 2018; 156: 328–351. doi: 10.1016/j.energy.2018.05.106
Theo WL, Lim JS, Hashim H, et al. Review of pre-combustion capture and ionic liquid in carbon capture and storage. Applied Energy 2016; 183: 1633–1663. doi: 10.1016/j.apenergy.2016.09.103
Zhu L, Jiang P, Fan J. Comparison of carbon capture IGCC with chemical-looping combustion and with calcium-looping process driven by coal for power generation. Chemical Engineering Research and Design 2015; 104: 110–124. doi: 10.1016/j.cherd.2015.07.027
Zhang Z, Borhani TNG, El-Naas MH. Carbon capture. In: Dincer I, Colpan CO, Kizilkan OBTE (editors). Energetic and Environmental Dimensions. Academic Press; 2018. pp. 997–1016.
Lee SY, Park SJ. A review on solid adsorbents for carbon dioxide capture. Journal of Industrial and Engineering Chemistry 2015; 23: 1–11. doi: 10.1016/j.jiec.2014.09.001
Tamajón FJ, Álvarez E, Cerdeira F, et al. CO2 absorption into N-methyldiethanolamine aqueous-organic solvents. Chemical Engineering Journal 2016; 283: 1069–1080. doi: 10.1016/j.cej.2015.08.065
Mohr VH, Ranke G. Acid and sour gas treating processes. Chemical Engineering Progress 1984; 80(10): 27–34.
Kapetaki Z, Brandani P, Brandani S, et al. Process simulation of a dual-stage Selexol process for 95% carbon capture efficiency at an integrated gasification combined cycle power plant. International Journal of Greenhouse Gas Control 2015; 39: 17–26. doi: 10.1016/j.ijggc.2015.04.015
Ban ZH, Keong LK, Mohd Shariff A. Physical absorption of CO2 capture: A review. Advanced Materials Research 2014; 917: 134–143. doi: 10.4028/www.scientific.net/AMR.917.134
Ahn H. Process Simulation of a dual-stage selexol process for pre-combustion carbon capture at an integrated gasification combined cycle power plant. In: Papadopoulos AI, Seferlis P (editors). Process Systems and Materials for CO2 Capture: Modelling, Design, Control and Integration. Wiley; 2017. pp. 609–628. doi: 10.1002/9781119106418.ch242017:
Gatti M, Martelli E, Maréchal F, Consonnid S. Multi-objective optimization of a Selexol® process for the selective removal of CO2 and H2S from coal-derived syngas. In: Proceedings of ECOS 2015 – The 28th International Conference on Efficiency, Cost, Optimization, Simulation and Environmental Impact of Energy System; 30 Jun–3 July 2015; Pau, France. pp. 1–13.
Im D, Roh K, Kim J, et al. Economic assessment and optimization of the Selexol process with novel additives. International Journal of Greenhouse Gas Control 2015; 42: 109–116. doi: 10.1016/j.ijggc.2015.08.001
Sun L, Smith R. Rectisol wash process simulation and analysis. Journal of Cleaner Production 2013; 39: 321–328. doi: 10.1016/j.jclepro.2012.05.049
Sharma I, Hoadley AFA, Mahajani SM, et al. Multi-objective optimisation of a Rectisol™ process for carbon capture. Journal of Cleaner Production 2016; 119: 196–206. doi: 10.1016/j.jclepro.2016.01.078
Gao N, Zhai C, Sun W, et al. Equation oriented method for Rectisol wash modeling and analysis. Chinese Journal of Chemical Engineering 2015; 23(9): 1530–1535. doi: 10.1016/j.cjche.2015.03.010
Nunes AVM, Carrera GVSM, Najdanovic-Visak V, et al. Solubility of CO2 in glycerol at high pressures. Fluid Phase Equilibria 2013; 358: 105–107. doi: 10.1016/j.fluid.2013.07.051
Kortunov PV, Siskin M, Baugh LS, et al. In situ nuclear magnetic resonance mechanistic studies of carbon dioxide reactions with liquid amines in aqueous systems: New insights on carbon capture reaction pathways. Energy & Fuels 2015; 29(9): 5919–5939. doi: 10.1021/acs.energyfuels.5b00985
Ochedi FO, Yu J, Yu H, et al. Carbon dioxide capture using liquid absorption methods: A review. Environmental Chemistry Letters 2021; 19: 77–109. doi: 10.1007/s10311-020-01093-8
Stöver B, Bergins C, Klebes J. Optimized post combustion carbon capturing on coal fired power plants. Energy Procedia 2011; 4: 1637–1643. doi: 10.1016/j.egypro.2011.02.035
Tobiesen FA, Svendsen HF. Study of a modified amine-based regeneration unit. Industrial & Engineering Chemistry Research 2006; 45(8): 2489–2496. doi: 10.1021/ie050544f
Ghayur A, Verheyen TV, Meuleman E. Biological and chemical treatment technologies for waste amines from CO2 capture plants. Journal of Environmental Management 2019; 241: 514–524. doi: 10.1016/j.jenvman.2018.07.033
Zhang S, Shen Y, Wang L, et al. Phase change solvents for post-combustion CO2 capture: Principle, advances, and challenges. Applied Energy 2019; 239: 876–897. doi: 10.1016/j.apenergy.2019.01.242
Ochedi FO, Yu J, Yu H, et al. Carbon dioxide capture using liquid absorption methods: A review. Environmental Chemistry Letters 2021; 19: 77–109. doi: 10.1007/s10311-020-01093-8
Bottoms RR. Separating acid gases, Girdler Corp. U.S. Patent 1,783,901, 2 December 1930.
Nwaoha C, Supap T, Idem R, et al. Advancement and new perspectives of using formulated reactive amine blends for post-combustion carbon dioxide (CO2) capture technologies. Petroleum 2017; 3(1): 10–36. doi: 10.1016/j.petlm.2016.11.002
Xue B, Yu Y, Chen J, et al. A comparative study of MEA and DEA for post-combustion CO2 capture with different process configurations. International Journal of Coal Science & Technology 2017; 4: 15–24. doi: 10.1007/s40789-016-0149-7
Shunji K, Xizhou S, Wenze Y. Investigation of CO2 desorption kinetics in MDEA and MDEA + DEA rich amine solutions with thermo-gravimetric analysis method. International Journal of Greenhouse Gas Control 2020; 95: 102947. doi: 10.1016/j.ijggc.2019.102947
Rodríguez N, Mussati S, Scenna N. Optimization of post-combustion CO2 process using DEA–MDEA mixtures. Chemical Engineering Research and Design 2011; 89(9): 1763–1773. doi: 10.1016/j.cherd.2010.11.009
Bajpai A, Mondal MK. Equilibrium solubility of CO2 in aqueous mixtures of DEA and AEEA. Journal of Chemical & Engineering Data 2013; 58(6): 1490–1495. doi: 10.1021/je3011776
Abd AA, Naji SZ, Barifcani A. Comprehensive evaluation and sensitivity analysis of regeneration energy for acid gas removal plant using single and activated-methyl diethanolamine solvents. Chinese Journal of Chemical Engineering 2020; 28(6): 1684–1693. doi: 10.1016/j.cjche.2019.12.004
Zhang Y, Chen CC. Thermodynamic modeling for CO2 absorption in aqueous MDEA solution with electrolyte NRTL model. Industrial & Engineering Chemistry Research 2011; 50(1): 163–175. doi: 10.1021/ie1006855
Xu F, Gao H, Dong H, et al. Solubility of CO2 in aqueous mixtures of monoethanolamine and dicyanamide-based ionic liquids. Fluid Phase Equilibria 2014; 365: 80–87. doi: 10.1016/j.fluid.2013.12.020
Sartori G, Savage DW. Sterically hindered amines for carbon dioxide removal from gases. Industrial & Engineering Chemistry Fundamentals 1983; 22(2): 239–249. doi: 10.1021/i100010a016
Sun WC, Yong CB, Li MH. Kinetics of the absorption of carbon dioxide into mixed aqueous solutions of 2-amino-2-methyl-l-propanol and piperazine. Chemical Engineering Science 2005; 60(2): 503–516. doi: 10.1016/j.ces.2004.08.012
Chakraborty AK, Astarita G, Bischoff KB. CO2 absorption in aqueous solutions of hindered amines. Chemical Engineering Science 1986; 41(4): 997–1003. doi: 10.1016/0009-2509(86)87185-8
Nwaoha C, Saiwan C, Tontiwachwuthikul P, et al. Carbon dioxide (CO2) capture: Absorption-desorption capabilities of 2-amino-2-methyl-1-propanol (AMP), piperazine (PZ) and monoethanolamine (MEA) tri-solvent blends. Journal of Natural Gas Science and Engineering 2016; 33: 742–750. doi: 10.1016/j.jngse.2016.06.002
Chen S, Han X, Sun X, et al. The comparative kinetics study of CO2 absorption into non-aqueous DEEA/MEA and DMEA/MEA blended systems solution by using stopped-flow technique. Chemical Engineering Journal 2020; 386: 121295. doi: 10.1016/j.cej.2019.03.171
Chen S, Han X, Sun X, et al. The comparative kinetics study of CO2 absorption into non-aqueous DEEA/MEA and DMEA/MEA blended systems solution by using stopped-flow technique. Chemical Engineering Journal 2020; 386: 121295. doi: 10.1016/j.cej.2019.03.171
Brúder P, Grimstvedt A, Mejdell T, et al. CO2 capture into aqueous solutions of piperazine activated 2-amino-2-methyl-1-propanol. Chemical Engineering Science 2011; 66(23): 6193–6198. doi: 10.1016/j.ces.2011.08.051
Seo DJ, Hong WH. Effect of piperazine on the kinetics of carbon dioxide with aqueous solutions of 2-amino-2-methyl-1-propanol. Industrial & Engineering Chemistry Research 2000; 39(6): 2062–2067. doi: 10.1021/ie990846f
Yang ZY, Soriano AN, Caparanga AR, et al. Equilibrium solubility of carbon dioxide in (2-amino-2-methyl-1-propanol + piperazine + water). The Journal of Chemical Thermodynamics 2010; 42(5): 659–665. doi: 10.1016/j.jct.2009.12.006
Lawal O, Bello A, Idem R. The role of methyl diethanolamine (MDEA) in preventing the oxidative degradation of CO2 loaded and concentrated aqueous monoethanolamine (MEA)−MDEA blends during CO2 absorption from flue gases. Industrial & Engineering Chemistry Research 2005; 44(6): 1874–1896. doi: 10.1021/ie049261y
Zhao B, Liu F, Cui Z, et al. Enhancing the energetic efficiency of MDEA/PZ-based CO2 capture technology for a 650 MW power plant: Process improvement. Applied Energy 2017; 185: 362–375. doi: 10.1016/j.apenergy.2016.11.009
Zhang R, Zhang X, Yang Q, et al. Analysis of the reduction of energy cost by using MEA-MDEA-PZ solvent for post-combustion carbon dioxide capture (PCC). Applied Energy 2017; 205: 1002–1011. doi: 10.1016/j.apenergy.2017.08.130
Nwaoha C, Saiwan C, Supap T, et al. Carbon dioxide (CO2) capture performance of aqueous tri-solvent blends containing 2-amino-2-methyl-1-propanol (AMP) and methyldiethanolamine (MDEA) promoted by diethylenetriamine (DETA). International Journal of Greenhouse Gas Control 2016; 53: 292–304. doi: 10.1016/j.ijggc.2016.08.012
Liu Y, Fan W, Wang K, et al. Studies of CO2 absorption/regeneration performances of novel aqueous monothanlamine (MEA)-based solutions. Journal of Cleaner Production 2016; 112: 4012–4021. doi: 10.1016/j.jclepro.2015.08.116
Akachuku A, Osei P A, Decardi-Nelson B, et al. Experimental and kinetic study of the catalytic desorption of CO2 from CO2-loaded monoethanolamine (MEA) and blended monoethanolamine–Methyl-diethanolamine (MEA-MDEA) solutions. Energy 2019; 179: 475–489. doi: 10.1016/j.energy.2019.04.174
Rahimi K, Riahi S, Abbasi M. Effect of host fluid and hydrophilicity of multi-walled carbon nanotubes on stability and CO2 absorption of amine-based and water-based nanofluids. Journal of Environmental Chemical Engineering 2020; 8(1): 103580. doi: 10.1016/J.JECE.2019.103580
Lai Q, Toan S, Assiri MA, et al. Catalyst-TiO (OH)2 could drastically reduce the energy consumption of CO2 capture. Nature Communications 2018; 9(1): 2672. doi: 10.1038/s41467-018-05145-0
Lee WY, Park SY, Lee KB, et al. Simultaneous removal of CO2 and H2S from biogas by blending amine absorbents: A performance comparison study. Energy & Fuels 2020; 34(2): 1992–2000. doi: 10.1021/acs.energyfuels.9b03342
Ashraf MA, Liu Z, Li C, et al. Assessment of mass transfer correlations used in post-combustion CO2 capture by piperazine activated 2-amino-2-methyl-1-propanol (a-AMP). Journal of Natural Gas Science and Engineering 2020; 73: 103051. doi: 10.1016/j.jngse.2019.103051
Kang MK, Jeon SB, Cho JH, et al. Characterization and comparison of the CO2 absorption performance into aqueous, quasi-aqueous and non-aqueous MEA solutions. International Journal of Greenhouse Gas Control 2017; 63: 281–288. doi: 10.1016/j.ijggc.2017.05.020
Xiao M, Liu H, Gao H, et al. CO2 absorption with aqueous tertiary amine solutions: Equilibrium solubility and thermodynamic modeling. The Journal of Chemical Thermodynamics 2018; 122: 170–182. doi: 10.1016/j.jct.2018.03.020
Luo X, Liu S, Gao H, et al. An improved fast screening method for single and blended amine-based solvents for post-combustion CO2 capture. Separation and Purification Technology 2016; 169: 279–288. doi: 10.1016/j.seppur.2016.06.018
Leimbrink M, Sandkämper S, Wardhaugh L, et al. Energy-efficient solvent regeneration in enzymatic reactive absorption for carbon dioxide capture. Applied Energy 2017; 208: 263–276. doi: 10.1016/j.apenergy.2017.10.042
Dey A, Dash SK, Mandal B. Equilibrium CO2 solubility and thermophysical properties of aqueous blends of 1-(2-aminoethyl) piperazine and N-methyldiethanolamine. Fluid Phase Equilibria 2018; 463: 91–105. doi: 10.1016/j.fluid.2018.01.030
Knuutila HK, Nannestad Å. Effect of the concentration of MAPA on the heat of absorption of CO2 and on the cyclic capacity in DEEA-MAPA blends. International Journal of Greenhouse Gas Control 2017; 61: 94–103. doi: 10.1016/j.ijggc.2017.03.026
Raynal L, Alix P, Bouillon PA, et al. The DMX™ process: An original solution for lowering the cost of post-combustion carbon capture. Energy Procedia 2011; 4: 779–786. doi: 10.1016/j.egypro.2011.01.119
Zhang W, Jin X, Tu W, et al. A novel CO2 phase change absorbent: MEA/1-propanol/H2O. Energy & Fuels 2017; 31(4): 4273–4279. doi: 10.1021/acs.energyfuels.7b00090
Wang L, Zhang Y, Wang R, et al. Advanced monoethanolamine absorption using sulfolane as a phase splitter for CO2 capture. Environmental Science & Technology 2018; 52(24): 14556–14563. doi: 10.1021/acs.est.8b05654
Wang L, Liu S, Wang R, Li Q, Zhang S. Regulating phase separation behavior of a DEEA–TETA biphasic solvent using sulfolane for energy-saving CO2 capture. Environmental Science & Technology 2019; 53(21): 12873–12881. doi: 10.1021/acs.est.9b02787
Barzagli F, Lai S, Mani F, et al. Novel non-aqueous amine solvents for biogas upgrading. Energy & Fuels 2014; 28(8): 5252–5258. doi: 10.1021/ef501170d
Bougie F, Pokras D, Fan X. Novel non-aqueous MEA solutions for CO2 capture. International Journal of Greenhouse Gas Control 2019; 86: 34–42. doi: 10.1016/j.ijggc.2019.04.013
Wang R, Jiang L, Li Q, et al. Energy-saving CO2 capture using sulfolane-regulated biphasic solvent. Energy 2020; 211: 118667. doi: 10.1016/j.energy.2020.118667
Zhuang Q, Clements B. CO2 capture by biphasic absorbent–absorption performance and VLE characteristics. Energy 2018; 147: 169–176. doi: 10.1016/j.energy.2018.01.004
Li X, Zhou X, Wei J, et al. Reducing the energy penalty and corrosion of carbon dioxide capture using a novel nonaqueous monoethanolamine-based biphasic solvent. Separation and Purification Technology 2021; 265: 118481. doi: 10.1016/j.seppur.2021.118481
Tao M, Gao J, Zhang W, et al. A novel phase-changing nonaqueous solution for CO2 capture with high capacity, thermostability, and regeneration efficiency. Industrial & Engineering Chemistry Research 2018; 57(28): 9305–9312. doi: 10.1021/acs.iecr.8b01775
Wang R, Liu S, Wang L, et al. Superior energy-saving splitter in monoethanolamine-based biphasic solvents for CO2 capture from coal-fired flue gas. Applied Energy 2019; 242: 302–310. doi: 10.1016/j.apenergy.2019.03.138
Wang R, Yang Y, Wang M, et al. Energy efficient diethylenetriamine–1-propanol biphasic solvent for CO2 capture: Experimental and theoretical study. Applied Energy 2021; 290: 116768. doi: 10.1016/j.apenergy.2021.116768
Zhou X, Li X, Wei J, et al. Novel nonaqueous liquid–liquid biphasic solvent for energy-efficient carbon dioxide capture with low corrosivity. Environmental Science & Technology 2020; 54(24): 16138–16146. doi: 10.1021/acs.est.0c05774
Li Y, Cheng J, Hu L, et al. Phase-changing solution PZ/DMF for efficient CO2 capture and low corrosiveness to carbon steel. Fuel 2018; 216: 418–426. doi: 10.1016/j.fuel.2017.12.030
Gao X, Li X, Cheng S, et al. A novel solid-liquid ‘phase controllable’ biphasic amine absorbent for CO2 capture. Chemical Engineering Journal 2022; 430: 132932. doi: 10.1016/j.cej.2021.132932
Bhatti UH, Shah AK, Kim JN, et al. Effects of transition metal oxide catalysts on MEA solvent regeneration for the post-combustion carbon capture process. ACS Sustainable Chemistry & Engineering 2017; 5(7): 5862–5868. doi: 10.1021/acssuschemeng.7b00604
Bhatti UH, Shah AK, Hussain A, et al. Catalytic activity of facilely synthesized mesoporous HZSM-5 catalysts for optimizing the CO2 desorption rate from CO2-rich amine solutions. Chemical Engineering Journal 2020; 389: 123439. doi: 10.1016/j.cej.2019.123439
Chen S, Chen S, Zhang Y, et al. Species distribution of CO2 absorption/desorption in aqueous and non-aqueous N-ethylmonoethanolamine solutions. International Journal of Greenhouse Gas Control 2016, 47: 151–158. doi: 10.1016/j.ijggc.2016.01.046
Liu F, Jing G, Zhou X, et al. Performance and mechanisms of triethylene tetramine (TETA) and 2-amino-2-methyl-1-propanol (AMP) in aqueous and nonaqueous solutions for CO2 capture. ACS Sustainable Chemistry & Engineering 2018; 6(1): 1352–1361. doi: 10.1021/acssuschemeng.7b03717
Ulus N, Syed Ali SA, Khalifa O, et al. Optimization of novel nonaqueous hexanol-based monoethanolamine/methyl diethanolamine solvent for CO2 absorption. International Journal of Energy Research 2022; 46(7): 9000–9019. doi: 10.1002/er.7779
Barbarossa V, Barzagli F, Mani F, et al. Efficient CO2 capture by non-aqueous 2-amino-2-methyl-1-propanol (AMP) and low temperature solvent regeneration. RSC Advances 2013; 3(30): 12349–12355. doi: 10.1039/c3ra40933c
Yang D, Lv M, Chen J. Efficient non-aqueous solvent formed by 2-piperidineethanol and ethylene glycol for CO2 absorption. Chemical Communications 2019; 55(83): 12483–12486. doi: 10.1039/c9cc06320j
Zheng C, Tan J, Wang Y J, et al. CO2 solubility in a mixture absorption system of 2-amino-2-methyl-1-propanol with ethylene glycol. Industrial & Engineering Chemistry Research 2013; 52(34): 12247–12252. doi: 10.1021/ie401805n
Li J, You C, Chen L, et al. Dynamics of CO2 absorption and desorption processes in alkanolamine with cosolvent polyethylene glycol. Industrial & Engineering Chemistry Research 2012; 51(37): 12081–12088. doi: 10.1021/ie301164v
Tian W, Ma K, Ji J, et al. Nonaqueous MEA/PEG200 absorbent with high efficiency and low energy consumption for CO2 capture. Industrial & Engineering Chemistry Research 2021; 60(10): 3871–3880. doi: 10.1021/acs.iecr.0c05294
Guo H, Li C, Shi X, et al. Nonaqueous amine-based absorbents for energy efficient CO2 capture. Applied Energy 2019; 239: 725–734. doi: 10.1016/j.apenergy.2019.02.019
Barzagli F, Giorgi C, Mani F, et al. Reversible carbon dioxide capture by aqueous and non-aqueous amine-based absorbents: A comparative analysis carried out by 13C NMR spectroscopy. Applied Energy 2018; 220: 208–219. doi: 10.1016/j.apenergy.2018.03.076
Barzagli F, Lai S, Mani F. Novel non-aqueous amine solvents for reversible CO2 capture. Energy Procedia 2014; 63: 1795–1804. doi: 10.1016/j.egypro.2014.11.186
Khan SN, Hailegiorgis SM, Man Z, et al. Thermophysical properties of concentrated aqueous solution of N-methyldiethanolamine (MDEA), piperazine (PZ), and ionic liquids hybrid solvent for CO2 capture. Journal of Molecular Liquids 2017; 229: 221–229. doi: 10.1016/j.molliq.2016.12.056
Yang J, Yu X, An L, et al. CO2 capture with the absorbent of a mixed ionic liquid and amine solution considering the effects of SO2 and O2. Applied Energy 2017; 194: 9–18. doi: 10.1016/j.apenergy.2017.02.071
Xiao M, Liu H, Gao H, et al. CO2 capture with hybrid absorbents of low viscosity imidazolium-based ionic liquids and amine. Applied Energy 2019; 235: 311–319. doi: 10.1016/j.apenergy.2018.10.103
Li J, Li Y, Li C, et al. CO2 absorption and microwave regeneration with high-concentration TETA nonaqueous absorbents. Greenhouse Gases: Science and Technology 2022; 12(3): 362–375. doi: 10.1002/ghg.2148
Li Y, Gao J, Li J, et al. Screening and performance evaluation of triethylenetetramine nonaqueous solutions for CO2 capture with microwave regeneration. Energy & Fuels 2020; 34(9): 11270–11281. doi: 10.1021/acs.energyfuels.0c02006
Yu CH, Wu TW, Tan CS. CO2 capture by piperazine mixed with non-aqueous solvent diethylene glycol in a rotating packed bed. International Journal of Greenhouse Gas Control 2013; 19: 503–509. doi: 10.1016/j.ijggc.2013.10.014
Chen M, Li M, Zhang F, et al. Fast and efficient CO2 absorption in non-aqueous tertiary amines promoted by ethylene glycol. Energy & Fuels 2022; 36(9): 4830–4836. doi: 10.1021/acs.energyfuels.2c00215
Bihong L, Kexuan Y, Xiaobin Z, et al. 2-Amino-2-methyl-1-propanol based non-aqueous absorbent for energy-efficient and non-corrosive carbon dioxide capture. Applied Energy 2020; 264: 114703. doi: 10.1016/j.apenergy.2020.114703
Darde V, Van Well WJM, Fosboel PL, et al. Experimental measurement and modeling of the rate of absorption of carbon dioxide by aqueous ammonia. International Journal of Greenhouse Gas Control 2011; 5(5): 1149–1162. doi: 10.1016/j.ijggc.2011.07.008
Wang F, Zhao J, Miao H, et al. Current status and challenges of the ammonia escape inhibition technologies in ammonia-based CO2 capture process. Applied Energy 2018; 230: 734–749. doi: 10.1016/j.apenergy.2018.08.116
Kohl AL, Riesenfeld FC. Alkaline salt solutions for hydrogen sulfide and carbon dioxide absorption. In: Gas Purification. Gulf Publishing Company; 1985. pp. 211–246.
Benson HE, Field JH, Jimeson RM. CO2 absorption: Employing hot potassium carbonate solutions. Chemical Engineering Progress (United States) 1954; 50(7).
Thee H, Suryaputradinata YA, Mumford KA, et al. A kinetic and process modeling study of CO2 capture with MEA-promoted potassium carbonate solutions. Chemical Engineering Journal 2012; 210: 271–279. doi: 10.1016/j.cej.2012.08.092
Bhosale RR, Kumar A, AlMomani F, et al. CO2 capture using aqueous potassium carbonate promoted by ethylaminoethanol: A kinetic study. Industrial & Engineering Chemistry Research 2016; 55(18): 5238–5246. doi: 10.1021/acs.iecr.5b04398
Mumford KA, Wu Y, Smith KH, Stevens GW. Review of solvent based carbon-dioxide capture technologies. Frontiers of Chemical Science and Engineering 2015; 9(2): 125–141. doi: 10.1007/s11705-015-1514-6
Smith KH, Anderson CJ, Tao W, et al. Pre-combustion capture of CO2—Results from solvent absorption pilot plant trials using 30 wt% potassium carbonate and boric acid promoted potassium carbonate solvent. International Journal of Greenhouse Gas Control 2012; 10: 64–73. doi: 10.1016/j.ijggc.2012.05.018
Berrouk AS, Ochieng R. Improved performance of the natural-gas-sweetening Benfield-HiPure process using process simulation. Fuel Processing Technology 2014; 127: 20–25. doi: 10.1016/j.fuproc.2014.06.012
Borhani TNG, Akbari V, Hamid MKA, et al. Rate-based simulation and comparison of various promoters for CO2 capture in industrial DEA-promoted potassium carbonate absorption unit. Journal of Industrial and Engineering Chemistry 2015; 22: 306–316. doi: 10.1016/j.jiec.2014.07.024
Borhani TNG, Azarpour A, Akbari V, et al. CO2 capture with potassium carbonate solutions: A state-of-the-art review. International Journal of Greenhouse Gas Control 2015; 41: 142–162. doi: 10.1016/j.ijggc.2015.06.026
Valluri S, Kawatra SK. Use of frothers to improve the absorption efficiency of dilute sodium carbonate slurry for post combustion CO2 capture. Fuel Processing Technology 2021; 212: 106620. doi: 10.1016/j.fuproc.2020.106620
Huang HP, Shi Y, Li W, et al. Dual alkali approaches for the capture and separation of CO2. Energy & Fuels 2001; 15(2): 263–268. doi: 10.1021/ef0002400
Vaezi MJ, Kojabad ME, Beiragh MM, et al. Transport mechanism and modeling of microporous zeolite membranes. In: Current Trends and Future Developments on (Bio-) Membranes. Elsevier; 2019. pp. 185–203.
Wang M, Zhao J, Wang X, et al. Recent progress on submicron gas-selective polymeric membranes. Journal of Materials Chemistry A 2017; 5(19): 8860–8886. doi: 10.1039/C7TA01862B
Zarca G, Horne WJ, Ortiz I, et al. Synthesis and gas separation properties of poly (ionic liquid)-ionic liquid composite membranes containing a copper salt. Journal of Membrane Science 2016; 515: 109–114. doi: 10.1016/J.MEMSCI.2016.05.045
Berstad D, Anantharaman R, Nekså P. Low-temperature CCS from an IGCC power plant and comparison with physical solvents. Energy Procedia 2013; 37: 2204–2211. doi: 10.1016/j.egypro.2013.06.100
Favre E. Carbon dioxide recovery from post-combustion processes: Can gas permeation membranes compete with absorption? Journal of Membrane Science 2007; 294(1–2): 50–59. doi: 10.1016/j.memsci.2007.02.007
D’Alessandro DM, Smit B, Long J R. Carbon dioxide capture: Prospects for new materials. Angewandte Chemie International Edition 2010; 49(35): 6058–6082. doi: 10.1002/anie.201000431
Ünveren EE, Monkul BÖ, Sarıoğlan Ş, et al. Solid amine sorbents for CO2 capture by chemical adsorption: A review. Petroleum 2017; 3(1): 37–50. doi: 10.1016/j.petlm.2016.11.001
Lai JY, Ngu LH, Hashim SS. A review of CO2 adsorbents performance for different carbon capture technology processes conditions. Greenhouse Gases: Science and Technology 2021; 11(5): 1076–1117. doi: 10.1002/ghg.2112
Ghanbari T, Abnisa F, Daud WMAW. A review on production of metal organic frameworks (MOF) for CO2 adsorption. Science of The Total Environment 2020; 707: 135090. doi: 10.1016/j.scitotenv.2019.135090
Kolle JM, Fayaz M, Sayari A. Understanding the effect of water on CO2 adsorption. Chemical Reviews 2021; 121(13): 7280–7345. doi: 10.1021/acs.chemrev.0c00762
Petrovic B, Gorbounov M, Soltani SM. Influence of surface modification on selective CO2 adsorption: A technical review on mechanisms and methods. Microporous and Mesoporous Materials 2021; 312: 110751. doi: 10.1016/j.micromeso.2020.110751
Wang Q, Luo J, Zhong Z, et al. CO2 capture by solid adsorbents and their applications: Current status and new trends. Energy & Environmental Science 2011; 4(1): 42–55. doi: 10.1039/C0EE00064G
Aghel B, Janati S, Wongwises S, et al. Review on CO2 capture by blended amine solutions. International Journal of Greenhouse Gas Control 2022; 119: 103715. doi: 10.1016/j.ijggc.2022.103715
Palomar J, Larriba M, Lemus J, et al. Demonstrating the key role of kinetics over thermodynamics in the selection of ionic liquids for CO2 physical absorption. Separation and Purification Technology 2019; 213: 578–586. doi: 10.1016/j.seppur.2018.12.059
Siriwardane RV, Shen MS, Fisher EP, et al. Adsorption of CO2 on molecular sieves and activated carbon. Energy & Fuels 2001; 15(2): 279–284. doi: 10.1021/ef000241s
Sayari A, Belmabkhout Y, Serna-Guerrero R. Flue gas treatment via CO2 adsorption. Chemical Engineering Journal 2011; 171(3): 760–774. doi: 10.1016/j.cej.2011.02.007
Chaffee A L, Knowles GP, Liang Z, et al. CO2 capture by adsorption: materials and process development. International Journal of Greenhouse Gas Control 2007; 1(1): 11–18. doi: 10.1016/S1750-5836(07)00031-X
Khalil SH, Aroua MK, Daud WMAW. Study on the improvement of the capacity of amine-impregnated commercial activated carbon beds for CO2 adsorbing. Chemical Engineering Journal 2012; 183: 15–20. doi: 10.1016/j.cej.2011.12.011
Perera MSA, Gamage RP, Rathnaweera TD, et al. A review of CO2-enhanced oil recovery with a simulated sensitivity analysis. Energies 2016; 9(7): 481. doi: 10.3390/en9070481
Panda M, Nottingham D, Lenig D. Systematic surveillance techniques for a large miscible WAG flood. SPE Reservoir Evaluation & Engineering 2011; 14(3): 299–309. doi: 10.2118/127563-MS
Branco CC. Challenges in implementing an EOR project in the pre-salt province in deep offshore Brasil. In: Proceedings of the SPE EOR Conference at Oil and Gas West Asia; April 2012; Muscat, Oman.
Ampomah W, Balch RS, Grigg RB, et al. Farnsworth field CO2-EOR project: Performance case history. In: Proceedings of the SPE Improved Oil Recovery Conference; April 2016; Tulsa, Oklahoma, USA.
Al Hajeri S, Negahban S, Al-Yafei G, et al. Design and Implementation of the first CO2-EOR Pilot in Abu Dhabi, UAE. In: Proceedings of the SPE EOR Conference at Oil and Gas West Asia; April 2010; Muscat, Oman.
Aresta M, Dibenedetto A, Angelini A. Catalysis for the valorization of exhaust carbon: From CO2 to chemicals, materials, and fuels. Technological use of CO2. Chemical Reviews 2014; 114(3): 1709–1742. doi: 10.1021/cr4002758
Hu B, Guild C, Suib SL. Thermal, electrochemical, and photochemical conversion of CO2 to fuels and value-added products. Journal of CO2 Utilization 2013; 1: 18–27. doi: 10.1016/j.jcou.2013.03.004
Fan MS, Abdullah AZ, Bhatia S. Catalytic technology for carbon dioxide reforming of methane to synthesis gas. ChemCatChem 2009; 1(2): 192–208. doi: 10.1002/cctc.200900025
Sanna A, Uibu M, Caramanna G, et al. A review of mineral carbonation technologies to sequester CO2. Chemical Society Reviews 2014; 43(23): 8049–8080. doi: 10.1039/C4CS00035H
Pan SY, Chiang A, Chang EE, et al. An innovative approach to integrated carbon mineralization and waste utilization: A review. Aerosol and Air Quality Research 2015; 15(3): 1072–1091. doi: 10.4209/aaqr.2014.10.0240
Bijl DL, Bogaart PW, Kram T, et al. Long-term water demand for electricity, industry and households. Environmental Science & Policy 2016; 55: 75–86. doi: 10.1016/j.envsci.2015.09.005ZZ
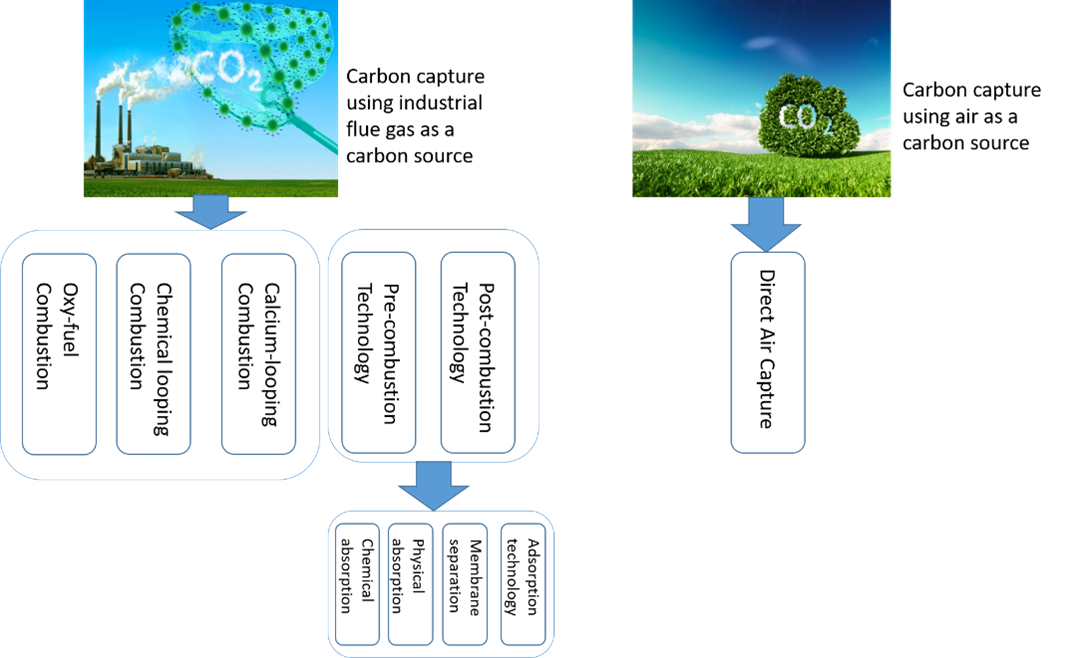
Downloads
Published
How to Cite
Issue
Section
License
Copyright (c) 2023 Guanchu Lu, Zhe Wang, Umair Hassan Bhatti, Xianfeng Fan

This work is licensed under a Creative Commons Attribution-NonCommercial 4.0 International License.