Possibility of the application of Si5O10–Ge5O10 for increasing H-adsorption towards the energy storage in transistors rather than Li-ion batteries
DOI:
https://doi.org/10.18686/cest286Keywords:
lithium battery; Li2(Si5O10–Ge5O10); Si5O10–Ge5O10; hydrogen storage; DFTAbstract
A comprehensive investigation on hydrogen grabbing via Si5O10–Ge5O10 was carried out including using density functional theory computations. The data showed that when silicon was replaced with germanium, the hydrogen-grabbing energy was ameliorated. The electromagnetic and thermodynamic properties of Si5O10–Ge5O10 and Li2(Si5O10–Ge5O10) nanoclusters were evaluated. The fluctuation in charge density values demonstrated that electronic densities were mainly located in the boundary of adsorbate/adsorbent atoms during adsorption. Therefore, it can be concluded that the Si5O10–Ge5O10 nanocluster might be an appropriate candidate for hydrogen storage in transistors. Lithium has an advantage over Si/Ge for possessing higher electron-and-hole motion, which allows lithium instruments to operate at higher frequencies than Si/Ge instruments.
References
1. Olabi AG, Sayed ET. Developments in Hydrogen Fuel Cells. Energies. 2023; 16(5): 2431. doi: 10.3390/en16052431 DOI: https://doi.org/10.3390/en16052431
2. Feng X, Sun L, Wang W, et al. Construction of CdS@ZnO core–shell nanorod arrays by atomic layer deposition for efficient photoelectrochemical H2 evolution. Separation and Purification Technology. 2023; 324: 124520. doi: 10.1016/j.seppur.2023.124520 DOI: https://doi.org/10.1016/j.seppur.2023.124520
3. Mollaamin F, Monajjemi M. In Silico-DFT Investigation of Nanocluster Alloys of Al-(Mg, Ge, Sn) Coated by Nitrogen Heterocyclic Carbenes as Corrosion Inhibitors. Journal of Cluster Science. 2023; 34: 2901-2918. doi: 10.1007/s10876-023-02436-5 DOI: https://doi.org/10.1007/s10876-023-02436-5
4. Das V, Padmanaban S, Venkitusamy K, et al. Recent advances and challenges of fuel cell based power system architectures and control–A review. Renewable and Sustainable Energy Reviews. 2017; 73: 10-18. doi: 10.1016/j.rser.2017.01.148 DOI: https://doi.org/10.1016/j.rser.2017.01.148
5. Lobo R, Ribeiro J, Inok F. Hydrogen Uptake and Release in Carbon Nanotube Electrocatalysts. Nanomaterials. 2021; 11(4): 975. doi: 10.3390/nano11040975 DOI: https://doi.org/10.3390/nano11040975
6. Wang Z, Li J, Hu C, et al. Hybrid energy storage system and management strategy for motor drive with high torque overload. Journal of Energy Storage. 2024; 75: 109432. doi: 10.1016/j.est.2023.109432 DOI: https://doi.org/10.1016/j.est.2023.109432
7. Strobel R, Garche J, Moseley PT, et al. Hydrogen Storage by Carbon Materials. Journal of Power Sources. 2006; 159(2): 781-801. doi: 10.1016/j.jpowsour.2006.03.04 DOI: https://doi.org/10.1016/j.jpowsour.2006.03.047
8. Baughman RH, Zakhidov AA, De Heer WA. Carbon Nanotubes–The Route toward Applications. Science. 2002; 297(5582): 787-792. doi: 10.1126/science.1060928 DOI: https://doi.org/10.1126/science.1060928
9. Fomkin A, Pribylov A, Men’shchikov I, et al. Adsorption-Based Hydrogen Storage in Activated Carbons and Model Carbon Structures. Reactions. 2021; 2(3): 209-226. doi: 10.3390/reactions2030014 DOI: https://doi.org/10.3390/reactions2030014
10. Zhao Y, Kim YH, Dillon AC, et al. Hydrogen Storage in Novel Organometallic Buckyballs. Physical Review Letters. 2005; 95: 155504. doi: 10.1103/PhysRevLett.94.155504 DOI: https://doi.org/10.1103/PhysRevLett.94.155504
11. Yang FH, Lachawiec AJ, Yang RT. Adsorption of Spillover Hydrogen Atoms on Single-Wall Carbon Nanotubes. The Journal of Physical Chemistry B. 2006; 110: 6236-6244. doi: 10.1021/jp056461u DOI: https://doi.org/10.1021/jp056461u
12. Novoselov KS, Geim AK, Morozov SV, et al. Electric field effect in atomically thin carbon films. Science. 2004; 306: 666-669. doi: 10.1126/science.1102896 DOI: https://doi.org/10.1126/science.1102896
13. Mollaamin F, Shahriari S, Monajjemi M, et al. Nanocluster of Aluminum Lattice via Organic Inhibitors Coating: A Study of Freundlich Adsorption. Journal of Cluster Science. 2023; 34: 1547-1562. doi: 10.1007/s10876-022-02335-1 DOI: https://doi.org/10.1007/s10876-022-02335-1
14. Castro Neto AH, Guinea F, Peres NMR, et al. The electronic properties of graphene. Review of Modern Physics. 2009; 81: 109-162. doi: 10.1103/RevModPhys.81.109 DOI: https://doi.org/10.1103/RevModPhys.81.109
15. Mak KF, Lee C, Hone J, et al. Atomically thin MoS2: A new direct-gap semiconductor. Physical Review Letters. 2010; 105: 136805-136807. doi: 10.1103/PhysRevLett.105.136805 DOI: https://doi.org/10.1103/PhysRevLett.105.136805
16. Zan L, Amin HMA, Mostafa E, et al. Electrodeposited cobalt nanosheets on smooth silver as a bifunctional catalyst for OER and ORR: In situ structural and catalytic characterization. ACS Applied Materials & Interfaces. 2022; 14: 55458-55470. doi: 10.1021/acsami.2c12163 DOI: https://doi.org/10.1021/acsami.2c12163
17. Zhao J, Kou M, Yuan Q, et al. Encapsulating Transition Metal Nanoparticles inside Carbon (TM@C) Chainmail Catalysts for Hydrogen Evolution Reactions: A Review. Molecules. 2024; 29: 4677. doi: 10.3390/molecules29194677 DOI: https://doi.org/10.3390/molecules29194677
18. Low T, Rodin AS, Carvalho A, et al. Tunable optical properties of multilayer black phosphorus thin films. Physical Review B. 2014; 90: 075434-074538. doi: 10.1103/PhysRevB.90.075434 DOI: https://doi.org/10.1103/PhysRevB.90.075434
19. Fei R, Faghaninia A, Soklaski R, et al. Enhanced thermoelectric efficiency via orthogonal electrical and thermal conductances in phosphorene. Nano Letters. 2014; 14(11): 6393-6399. doi: 10.1021/nl502865s DOI: https://doi.org/10.1021/nl502865s
20. Ramasubramaniam A, Muniz AR. Ab initio studies of thermodynamic and electronic properties of phosphorene nanoribbons. Physical Review B. 2014; 90: 085424-085429. doi: 10.1103/PhysRevB.90.085424 DOI: https://doi.org/10.1103/PhysRevB.90.085424
21. Yan Z, Bai Y, Sun L. Adsorption of thiophene and SOx molecules on Cr-doped and Ti-doped graphene nanosheets: A DFT study. Materials Research Express. 2019; 6(12): 125067. doi: 10.1088/2053-1591/ab599d DOI: https://doi.org/10.1088/2053-1591/ab599d
22. Mollaamin F, Monajjemi M. In Situ Ti-Embedded SiC as Chemiresistive Nanosensor for Safety Monitoring of CO, CO2, NO, NO2: Molecular Modelling by Conceptual Density Functional Theory. Russian Journal of Physical Chemistry B. 2024; 18: 49-66. doi: 10.1134/S1990793124010159 DOI: https://doi.org/10.1134/S1990793124010159
23. Javan MB. Electronic and magnetic properties of monolayer SiC sheet doped with 3d-transition metals. Journal of Magnetism and Magnetic Materials. 2016; 401: 656-661. doi: 10.1016/j.jmmm.2015.10.103 DOI: https://doi.org/10.1016/j.jmmm.2015.10.103
24. Li M, Liu Z, Wu D, et al. Transition Metal-Mediated Preparation of Nitrogen-Doped Porous Carbon for Advanced Zinc-Ion Hybrid Capacitors. Nanomaterials. 2025; 15: 83. doi: 10.3390/nano15020083 DOI: https://doi.org/10.3390/nano15020083
25. Wu Y, Zhou LP, Du XZ, Yang Y. Near-field radiative heat transfer between two SiC plates with/without coated metal films. Journal of Nanoscience and Nanotechnology. 2015; 15(4): 3017-3024. doi: 10.1166/jnn.2015.9687 DOI: https://doi.org/10.1166/jnn.2015.9687
26. Nazeer W, Farooq A, Younas M, et al. On Molecular Descriptors of Carbon Nanocones. Biomolecules. 2018; 8(3): 92. doi: 10.3390/biom8030092 DOI: https://doi.org/10.3390/biom8030092
27. Zhao J, Li Z, Cole MT, et al. Nanocone-Shaped Carbon Nanotubes Field-Emitter Array Fabricated by Laser Ablation. Nanomaterials. 2021; 11(12): 3244. doi: 10.3390/nano11123244 DOI: https://doi.org/10.3390/nano11123244
28. Rong Y, Cao Y, Guo N, et al. A simple method to synthesize V2O5 nanostructures with controllable morphology for high performance Li-ion batteries. Electrochimica Acta. 2016; 222: 1691-1699. doi: 10.1016/j.electacta.2016.11.160 DOI: https://doi.org/10.1016/j.electacta.2016.11.160
29. Zhu S, Jia H, Wang X, et al. Improved MRD 4H-SiC MESFET with High Power Added Efficiency. Micromachines. 2019; 10: 479. doi: 10.3390/mi10070479 DOI: https://doi.org/10.3390/mi10070479
30. Taha HO, El Mahdy AM, El Shemy FES, Hassan MM. Hydrogen storage in SiC, GeC, and SnC nanocones functionalized with nickel, Density Functional Theory—Study. International Journal of quantum Chemistry. 2023; 123(3): e27023. doi: 10.1002/qua.27023 DOI: https://doi.org/10.1002/qua.27023
31. Wei T, Zhou Y, Sun C. et al. An intermittent lithium deposition model based on CuMn-bimetallic MOF derivatives for composite lithium anode with ultrahigh areal capacity and current densities. Nano Research. 2023; 17: 2763-2769. doi: 10.1007/s12274-023-6187-8 DOI: https://doi.org/10.1007/s12274-023-6187-8
32. Thupsuri S, Tabtimsai C, Ruangpornvisuti V, Wanno B. A study of the transition metal doped boron nitride nanosheets as promising candidates for hydrogen and formaldehyde adsorptions. Physica E: Low-dimensional Systems and Nanostructures. 2021; 134: 114859. doi: 10.1016/j.physe.2021.114859 DOI: https://doi.org/10.1016/j.physe.2021.114859
33. Mollaamin F, Monajjemi M. Nanomaterials for Sustainable Energy in Hydrogen-Fuel Cell: Functionalization and Characterization of Carbon Nano-Semiconductors with Silicon, Germanium, Tin or Lead through Density Functional Theory Study. Russian Journal of Physical Chemistry B. 2024; 18: 607-623. doi: 10.1134/S1990793124020271 DOI: https://doi.org/10.1134/S1990793124020271
34. Monajjemi M, Mollaamin F. Development of Solid-State Lithium-Ion Batteries (LIBs) to Increase Ionic Conductivity through Interactions between Solid Electrolytes and Anode and Cathode Electrodes. Energies. 2024; 17(18): 4530. doi: 10.3390/en17184530 DOI: https://doi.org/10.3390/en17184530
35. Mollaamin F. Competitive Intracellular Hydrogen-nanocarrier Among Aluminum, Carbon, or Silicon Implantation: a Novel Technology of Eco-Friendly Energy Storage using Research Density Functional Theory. Russian Journal of Physical Chemistry B. 2024; 18: 805-820. doi: 10.1134/S1990793124700131 DOI: https://doi.org/10.1134/S1990793124700131
36. Bo Z, Guo X, Wei X, et al. Density functional theory calculations of NO2 and H2S adsorption on the group 10 transition metal (Ni, Pd and Pt) decorated graphene. Physica E: Low-dimensional Systems and Nanostructures. 2019; 109: 156-163. doi: 10.1016/j.physe.2019.01.012 DOI: https://doi.org/10.1016/j.physe.2019.01.012
37. Yi Y, Chen J, Zhao Y, et al. Large dielectric properties of niobate-titanate glass ceramics prepared by powder sintering method. Physica B: Condensed Matter. 2024; 694: 416462. doi: 10.1016/j.physb.2024.416462 DOI: https://doi.org/10.1016/j.physb.2024.416462
38. Li S, Tong L, Zhang B, Fu X. First-Principles Study of High-Entropy Sulfides and their Alkali Metal-Doped Modification as Cathode Material for Sodium-Ion Batteries. ChmePhysChem. 2024; 25(17): e202300999. doi: 10.1002/cphc.202300999 DOI: https://doi.org/10.1002/cphc.202300999
39. Guo X, Qin B, Guo Z, et al. Research on desalination performance of novel free-interface evaporation synergism membrane distillation module: Suitable for solar drive scenarios. Separation and Purification Technology. 2025; 361: 131350. doi: 10.1016/j.seppur.2024.131350 DOI: https://doi.org/10.1016/j.seppur.2024.131350
40. Jayanthi S, Vahini M, Karthickprabhu S, et al. The Transformative Role of Nano-SiO2 in Polymer Electrolytes for Enhanced Energy Storage Solutions. Processes. 2024; 12:2174. doi: 10.3390/pr12102174 DOI: https://doi.org/10.3390/pr12102174
41. Zolotarev KV, Mikhailov AN, Nakhod VI, et al. Characterization and in vivo toxicity assay of uncoated silicon nanoparticles. New Materials, Compounds and Applications. 2024; 8(2): 162-170. doi: 10.62476/nmca82162 DOI: https://doi.org/10.62476/nmca82162
42. Abdullayeva LK, Hasanov MH. Investigation of the role of metal in non-alloy metal-semiconductor (Si) contact. New Materials, Compounds and Applications. 2024; 8(1): 135-141. doi: 10.62476/nmca8135 DOI: https://doi.org/10.62476/nmca8135
43. Yanai T, Tew DP, Handy NC. A new hybrid exchange-correlation functional using the Coulomb-attenuating method (CAM-B3LYP), Chemical Physics Letters. 2004; 393(1-3): 51-57. doi: 10.1016/j.cplett.2004.06.011 DOI: https://doi.org/10.1016/j.cplett.2004.06.011
44. Grimme S, Antony J, Ehrlich S, Krieg H. A consistent and accurate ab initio parametrization of density functional dispersion correction (DFT-D) for the 94 elements H-Pu. The Journal of Chemical Physics. 2010; 132(15): 154104. doi: 10.1063/1.3382344 DOI: https://doi.org/10.1063/1.3382344
45. Grimme S, Ehrlich S, Goerigk L. Effect of the damping function in dispersion corrected density functional theory. Journal of Computational Chemistry. 2011; 32(7): 1456-1465. doi: 10.1002/jcc.21759 DOI: https://doi.org/10.1002/jcc.21759
46. Henkelman G, Arnaldsson A, Jónsson H. A fast and robust algorithm for Bader decomposition of charge density. Computational Materials Science. 2006; 36(3): 354-360. doi: 10.1016/j.commatsci.2005.04.010 DOI: https://doi.org/10.1016/j.commatsci.2005.04.010
47. Ritopečki MS, Skorodumova NV, Dobrota AS, Pašti IA. Density Functional Theory Analysis of the Impact of Boron Concentration and Surface Oxidation in Boron-Doped Graphene for Sodium and Aluminum Storage. C-Journal of Carbon Research. 2023; 9(4): 92. doi: 10.3390/c9040092 DOI: https://doi.org/10.3390/c9040092
48. Mollaamin F, Monajjemi M. Molecular modelling framework of metal-organic clusters for conserving surfaces: Langmuir sorption through the TD-DFT/ONIOM approach. Molecular Simulation. 2023; 49(4): 365-376. doi: 10.1080/08927022.2022.2159996 DOI: https://doi.org/10.1080/08927022.2022.2159996
49. Vosko SH, Wilk L, Nusair M. Accurate spin-dependent electron liquid correlation energies for local spin density calculations: A critical analysis. Canadian Journal of Physics. 1980; 58(8): 1200-1211. doi: 10.1139/p80-159 DOI: https://doi.org/10.1139/p80-159
50. Frisch MJ, Trucks GW, Schlegel HB, et al. Gaussian 16, Revision C.01. Available online: https://gaussian.com/ (accessed on 10 January 2025).
51. Dennington R, Keith TA, Millam JM. GaussView, Version 6.06.16. Available online: https://gaussian.com/gv6new/ (accessed on 10 January 2025).
52. Trontelj Z, Pirnat J, Jazbinšek V, et al. Nuclear Quadrupole Resonance (NQR)—A Useful Spectroscopic Tool in Pharmacy for the Study of Polymorphism. Crystals. 2020; 10(6): 450. doi: 10.3390/cryst10060450 DOI: https://doi.org/10.3390/cryst10060450
53. Sciotto R, Ruiz Alvarado IA, Schmidt WG. Substrate Doping and Defect Influence on P-Rich InP (001): H Surface Properties. Surfaces. 2024; 7(1): 79-87. doi: 10.3390/surfaces7010006 DOI: https://doi.org/10.3390/surfaces7010006
54. Luo J, Wang C, Wang Z, et al. NMR and NQR studies on transition-metal arsenide superconductors LaRu2As2, KCa2Fe4As4F2, and A2Cr3As3. Chinese Physics B. 2020; 29: 067402. doi: 10.1088/1674-1056/ab892d DOI: https://doi.org/10.1088/1674-1056/ab892d
55. Young HD, Freedman RA, Ford AL. Sears and Zemansky’s University Physics with Modern Physics, 13th ed. Addison-Wesley; 2012. p. 754.
56. Sohail U, Ullah F, Binti Zainal Arfan NH, et al. Transition Metal Sensing with Nitrogenated Holey Graphene: A First-Principles Investigation. Molecules. 2023; 28: 4060. doi: 10.3390/molecules28104060. DOI: https://doi.org/10.3390/molecules28104060
57. Sheet SHM, Mahmod RB, Saeed NHM, Saied SM. Theoretical study for comparison of pKa of a number of Schiff bases by employing parameters derived from DFT and MP2 method. New Materials, Compounds and Applications. 2024; 8(1): 94-108. doi: 10.62476/nmca8194 DOI: https://doi.org/10.62476/nmca8194
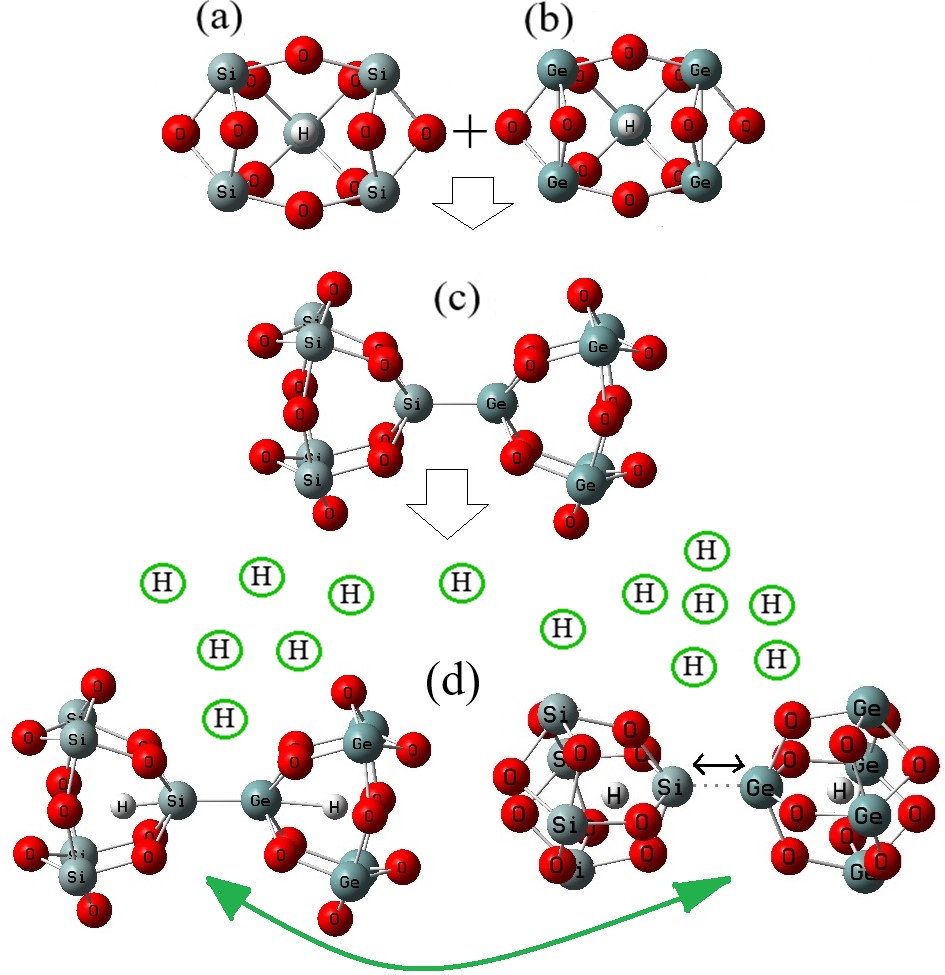
Downloads
Published
How to Cite
Issue
Section
License
Copyright (c) 2025 Fatemeh Mollaamin

This work is licensed under a Creative Commons Attribution 4.0 International License.