Investigation of hygrothermal behavior of a novel bio-based panel: Experiment and numerical simulation
DOI:
https://doi.org/10.18686/cest249Keywords:
straw composite; hygroscopic material; liquid water permeability; coupled heat and moisture transfer; inverse parameter estimationAbstract
Straw composites, owing to their low carbon footprint and favorable hygrothermal properties, are becoming a promising alternative insulation material for buildings in order to promote energy saving and occupants’ comfort. However, the heat and moisture characteristics of straw composites at the material scale and under steady-state condition are insufficient for a thorough assessment of their performance as a building component in actual service conditions. This study focused on the hygrothermal performance of a novel bio-based wall made with a rice straw–alginate composite material. The temperature and relative humidity profiles within the wall were monitored under various boundary conditions. The inverse analysis method was proposed to determine liquid water permeability. In in a dynamic test, compared with the model of coupled heat-and-moisture transfer (CHM), the transient heat transfer model predicted temperature profiles with higher errors and underestimated total heat flux by up to 30.6%. Also, under the dynamic condition, the CHM model with liquid water transport showed decreased mean absolute errors by 61%, 57% and 8% at depths of 28 mm, 36 mm and 64 mm, respectively, compared with those predicted by the CHM model without liquid water transport. Both vapor transport and liquid transport seemed to be essential when modeling thermal transfer and moisture transfer through the wall.
References
1. Chen Y, Thomas Ng S. Factoring in embodied GHG emissions when assessing the environmental performance of building. Sustainable Cities and Society. 2016; 27: 244–252. doi: 10.1016/j.scs.2016.03.015 DOI: https://doi.org/10.1016/j.scs.2016.03.015
2. Ramlee NA, Naveen J, Jawaid M. Potential of oil palm empty fruit bunch (OPEFB) and sugarcane bagasse fibers for thermal insulation application – A review. Construction and Building Materials. 2021; 271: 121519. doi: 10.1016/j.conbuildmat.2020.121519 DOI: https://doi.org/10.1016/j.conbuildmat.2020.121519
3. Muthuraj R, Lacoste C, Lacroix P, et al. Sustainable thermal insulation biocomposites from rice husk, wheat husk, wood fibers and textile waste fibers: Elaboration and performances evaluation. Industrial Crops and Products. 2019; 135: 238–245. doi: 10.1016/j.indcrop.2019.04.053 DOI: https://doi.org/10.1016/j.indcrop.2019.04.053
4. Mati-Baouche N, De Baynast H, Lebert A, et al. Mechanical, thermal and acoustical characterizations of an insulating bio-based composite made from sunflower stalks particles and chitosan. Industrial Crops and Products. 2014; 58: 244–250. doi: 10.1016/j.indcrop.2014.04.022 DOI: https://doi.org/10.1016/j.indcrop.2014.04.022
5. Nguyen DM, Grillet AC, Bui QB, et al. Building bio-insulation materials based on bamboo powder and bio-binders. Construction and Building Materials. 2018; 186: 686–698. doi: 10.1016/j.conbuildmat.2018.07.153 DOI: https://doi.org/10.1016/j.conbuildmat.2018.07.153
6. Viel M, Collet F, Lanos C. Development and characterization of thermal insulation materials from renewable resources. Construction and Building Materials. 2019; 214: 685–697. doi: 10.1016/j.conbuildmat.2019.04.139 DOI: https://doi.org/10.1016/j.conbuildmat.2019.04.139
7. Hassan SS, Williams GA, Jaiswal AK. Emerging technologies for the pretreatment of lignocellulosic biomass. Bioresource Technology. 2018; 262: 310–318. doi: 10.1016/j.biortech.2018.04.099 DOI: https://doi.org/10.1016/j.biortech.2018.04.099
8. Liuzzi S, Rubino C, Martellotta F, et al. Characterization of biomass-based materials for building applications: The case of straw and olive tree waste. Industrial Crops and Products. 2020; 147: 112229. doi: 10.1016/j.indcrop.2020.112229 DOI: https://doi.org/10.1016/j.indcrop.2020.112229
9. Dušek J, Jerman M, Podlena M, et al. Sustainable composite material based on surface-modified rape straw and environment-friendly adhesive. Construction and Building Materials. 2021; 300: 124036. doi: 10.1016/j.conbuildmat.2021.124036 DOI: https://doi.org/10.1016/j.conbuildmat.2021.124036
10. Rojas C, Cea M, Iriarte A, et al. Thermal insulation materials based on agricultural residual wheat straw and corn husk biomass, for application in sustainable buildings. Sustainable Materials and Technologies. 2019; 20: e00102. doi: 10.1016/j.susmat.2019.e00102 DOI: https://doi.org/10.1016/j.susmat.2019.e00102
11. Romano A, Bras A, Grammatikos S, et al. Dynamic behaviour of bio-based and recycled materials for indoor environmental comfort. Construction and Building Materials. 2019; 211: 730–743. doi: 10.1016/j.conbuildmat.2019.02.126 DOI: https://doi.org/10.1016/j.conbuildmat.2019.02.126
12. Ismail B, Belayachi N, Hoxha D. Hygric properties of wheat straw biocomposite containing natural additives intended for thermal insulation of buildings. Construction and Building Materials. 2022; 317: 126049. doi: 10.1016/j.conbuildmat.2021.126049 DOI: https://doi.org/10.1016/j.conbuildmat.2021.126049
13. Javaid A, Jain D, Kwatra N. Pre-treatment and its effect on the thermal conductivity of natural lignocellulosic rice straw stubble waste boards: Analysis vis a vis potential for the civil engineering applications. Construction and Building Materials. 2024; 445: 137903. doi: 10.1016/j.conbuildmat.2024.137903 DOI: https://doi.org/10.1016/j.conbuildmat.2024.137903
14. Thomson A, Walker P. Durability characteristics of straw bales in building envelopes. Construction and Building Materials. 2014; 68: 135–141. doi: 10.1016/j.conbuildmat.2014.06.041 DOI: https://doi.org/10.1016/j.conbuildmat.2014.06.041
15. Laborel-Préneron A, Ouédraogo K, Simons A, et al. Laboratory test to assess sensitivity of bio-based earth materials to fungal growth. Building and Environment. 2018; 142: 11–21. doi: 10.1016/j.buildenv.2018.06.003 DOI: https://doi.org/10.1016/j.buildenv.2018.06.003
16. Slimani Z, Trabelsi A, Virgone J, et al. Study of the Hygrothermal Behavior of Wood Fiber Insulation Subjected to Non-Isothermal Loading. Applied Sciences. 2019; 9(11): 2359. doi: 10.3390/app9112359 DOI: https://doi.org/10.3390/app9112359
17. Latif E, Lawrence RMH, Shea AD, et al. An experimental investigation into the comparative hygrothermal performance of wall panels incorporating wood fibre, mineral wool and hemp-lime. Energy and Buildings. 2018; 165: 76–91. doi: 10.1016/j.enbuild.2018.01.028 DOI: https://doi.org/10.1016/j.enbuild.2018.01.028
18. Seng B, Magniont C, Gallego S, et al. Behavior of a hemp-based concrete wall under dynamic thermal and hygric solicitations. Energy and Buildings. 2021; 232: 110669. doi: 10.1016/j.enbuild.2020.110669 DOI: https://doi.org/10.1016/j.enbuild.2020.110669
19. Chennouf N, Agoudjil B, Alioua T, et al. Experimental investigation on hygrothermal performance of a bio-based wall made of cement mortar filled with date palm fibers. Energy and Buildings. 2019; 202: 109413. doi: 10.1016/j.enbuild.2019.109413 DOI: https://doi.org/10.1016/j.enbuild.2019.109413
20. Rahim M, Douzane O, Tran Le AD, et al. Experimental investigation of hygrothermal behavior of two bio-based building envelopes. Energy and Buildings. 2017; 139: 608–615. doi: 10.1016/j.enbuild.2017.01.058 DOI: https://doi.org/10.1016/j.enbuild.2017.01.058
21. Alioua T, Agoudjil B, Chennouf N, et al. Investigation on heat and moisture transfer in bio-based building wall with consideration of the hysteresis effect. Building and Environment. 2019; 163: 106333. doi: 10.1016/j.buildenv.2019.106333 DOI: https://doi.org/10.1016/j.buildenv.2019.106333
22. Dong W, Chen Y, Bao Y, et al. A validation of dynamic hygrothermal model with coupled heat and moisture transfer in porous building materials and envelopes. Journal of Building Engineering. 2020; 32: 101484. doi: 10.1016/j.jobe.2020.101484 DOI: https://doi.org/10.1016/j.jobe.2020.101484
23. Kaoutari T, Louahlia H. Experimental and numerical investigations on the thermal and moisture transfer in green dual layer wall for building. Case Studies in Thermal Engineering. 2024; 53: 103946. doi: 10.1016/j.csite.2023.103946 DOI: https://doi.org/10.1016/j.csite.2023.103946
24. Mendes N, Winkelmann F, Lamberts R, Philippi PC. Moisture effects on conduction loads. Energy and buildings. 2003; 35(7): 631–644. DOI: https://doi.org/10.1016/S0378-7788(02)00171-8
25. Rouchier S, Busser T, Pailha M, et al. Hygric characterization of wood fiber insulation under uncertainty with dynamic measurements and Markov Chain Monte-Carlo algorithm. Building and Environment. 2017; 114: 129–139. doi: 10.1016/j.buildenv.2016.12.012 DOI: https://doi.org/10.1016/j.buildenv.2016.12.012
26. Dubois S, McGregor F, Evrard A, et al. An inverse modelling approach to estimate the hygric parameters of clay-based masonry during a Moisture Buffer Value test. Building and Environment. 2014; 81: 192–203. doi: 10.1016/j.buildenv.2014.06.018 DOI: https://doi.org/10.1016/j.buildenv.2014.06.018
27. Alioua T, Agoudjil B, Boudenne A, et al. Sensitivity analysis of transient heat and moisture transfer in a bio-based date palm concrete wall. Building and Environment. 2021; 202: 108019. doi: 10.1016/j.buildenv.2021.108019 DOI: https://doi.org/10.1016/j.buildenv.2021.108019
28. Zhou Y, Trabelsi A, El Mankibi M. Hygrothermal properties of insulation materials from rice straw and natural binders for buildings. Construction and Building Materials. 2023; 372: 130770. doi: 10.1016/j.conbuildmat.2023.130770 DOI: https://doi.org/10.1016/j.conbuildmat.2023.130770
29. Hagentoft CE. HAMSTAD—Final report: Methodology of HAM-modeling. Report R. 2002; 2 (8): 19–23.
30. Slimani Z. Experimental and numerical analysis of the hygrothermal behavior of highly hygroscopic walls (French). Lyon 1; 2015.
31. BS EN ISO 12571. Hygrothermal performance of building materials and products-Determination of hygroscopic sorption properties. British Standards Institution; 2013.
32. Künzel HM. Simultaneous heat and moisture transport in building components, One-and two-dimensional calculation using simple parameters. IRB-Verlag Stuttgart. 1995; 65.
33. Branco F, Tadeu A, Simões N. Heat conduction across double brick walls via BEM. Building and Environment. 2004; 39(1): 51–58. doi: 10.1016/j.buildenv.2003.08.005 DOI: https://doi.org/10.1016/j.buildenv.2003.08.005
34. Liu X, Chen Y, Ge H, et al. Numerical investigation for thermal performance of exterior walls of residential buildings with moisture transfer in hot summer and cold winter zone of China. Energy and Buildings. 2015; 93: 259–268. doi: 10.1016/j.enbuild.2015.02.016 DOI: https://doi.org/10.1016/j.enbuild.2015.02.016
35. Fang A, Chen Y, Wu L. Transient simulation of coupled heat and moisture transfer through multi-layer walls exposed to future climate in the hot and humid southern China area. Sustainable Cities and Society. 2020; 52: 101812. doi: 10.1016/j.scs.2019.101812 DOI: https://doi.org/10.1016/j.scs.2019.101812
36. Hagentoft CE, Kalagasidis AS, Adl-Zarrabi B, et al. Assessment Method of Numerical Prediction Models for Combined Heat, Air and Moisture Transfer in Building Components: Benchmarks for One-dimensional Cases. Journal of Thermal Envelope and Building Science. 2004; 27(4): 327–352. doi: 10.1177/1097196304042436 DOI: https://doi.org/10.1177/1097196304042436
37. Trabelsi A, Slimani Z, Virgone J. Response surface analysis of the dimensionless heat and mass transfer parameters of Medium Density Fiberboard. International Journal of Heat and Mass Transfer. 2018; 127: 623–630. doi: 10.1016/j.ijheatmasstransfer.2018.05.145 DOI: https://doi.org/10.1016/j.ijheatmasstransfer.2018.05.145
38. Eberhart R, Kennedy J. A new optimizer using particle swarm theory, Micro Mach, Hum. Sci; 1995.
39. Whitley D. A genetic algorithm tutorial. Statistics and Computing. 1994; 4(2): 65–85. doi: 10.1007/bf00175354 DOI: https://doi.org/10.1007/BF00175354
40. Chai T, Draxler RR. Root mean square error (RMSE) or mean absolute error (MAE), Geoscientific Model Development Discussions. 2014; 7(1): 1525–1534. doi: 10.5194/gmdd-7-1525-2014 DOI: https://doi.org/10.5194/gmdd-7-1525-2014
41. Asan H. Numerical computation of time lags and decrement factors for different building materials. Building and Environment. 2006; 41(5): 615–620. doi: 10.1016/j.buildenv.2005.02.020 DOI: https://doi.org/10.1016/j.buildenv.2005.02.020
42. Rafidiarison H, Mougel E, Nicolas A. Laboratory experiments on hygrothermal behaviour of real-scale timber walls. Maderas Ciencia y tecnología. 2012; (ahead): 0-0. doi: 10.4067/s0718-221x2012005000010 DOI: https://doi.org/10.4067/S0718-221X2012005000010
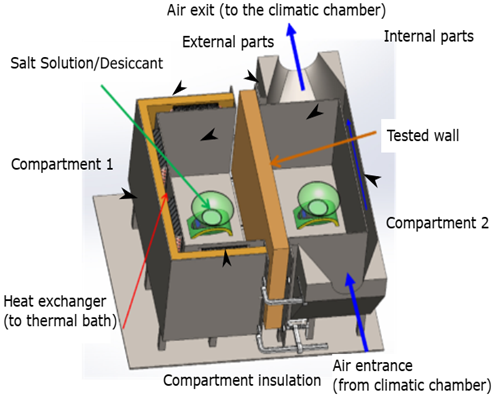
Downloads
Published
How to Cite
Issue
Section
License
Copyright (c) 2025 Yaping Zhou, Abdelkrim Trabelsi, Li Xiang, Mohamed El Mankibi

This work is licensed under a Creative Commons Attribution 4.0 International License.