Recent progress of MoS2 for photocatalytic and electrocatalytic hydrogen generation—A review
DOI:
https://doi.org/10.18686/cest.v2i3.157Keywords:
photocatalysis; electrocatalysis; hydrogen evolution reaction; molybdenum disulfideAbstract
Hydrogen (H2) plays a crucial role in the transformation of the energy structure due to its environmental friendliness, renewability and high energy density. The photocatalytic and electrocatalytic hydrogen evolution reaction (HER) presents a promising approach for H2 production. Molybdenum disulfide (MoS2) has emerged as a promising catalyst in photocatalytic and electrocatalytic HER due to its high activity, easy preparation and cheapness. However, it suffers from poor stability and inactive basal planes. In this review, we encapsulated the research advancements of MoS2 for photocatalytic and electrocatalytic HER in the past ~10 years. The latest strategies to enhance the catalytic activity of MoS2, such as doping, phase adjustment, surface modification and others, are also summarized. The relationship between structure and activity for enhanced H2 generation by different means is briefly introduced. The challenges and directions of MoS2 materials in photocatalysis and electrocatalysis for HER are also discussed, aiming to provide promising guidelines for future research.
References
1. Li R, Kuang P, Wageh S, et al. Potential-dependent reconstruction of Ni-based cuboid arrays for highly efficient hydrogen evolution coupled with electro-oxidation of organic compound. Chemical Engineering Journal. 2023; 453: 139797. doi: 10.1016/j.cej.2022.139797 DOI: https://doi.org/10.1016/j.cej.2022.139797
2. Lu G, Wang Z, Bhatti UH, et al. Recent progress in carbon dioxide capture technologies: A review. Clean Energy Science and Technology. 2023; 1(1). doi: 10.18686/cest.v1i1.32 DOI: https://doi.org/10.18686/cest.v1i1.32
3. Jiehui Z, Su Y, Wang W, et al. Hydrogen-electricity coupling energy storage systems: Models, applications, and deep reinforcement learning algorithms. Clean Energy Science and Technology. 2024; 2(1): 96. doi: 10.18686/cest.v2i1.96 DOI: https://doi.org/10.18686/cest.v2i1.96
4. Zhang Y, Xiao Y, Abuelgasim S, et al. A brief review of hydrogen production technologies. Clean Energy Science and Technology. 2024; 2(1): 117. doi: 10.18686/cest.v2i1.117 DOI: https://doi.org/10.18686/cest.v2i1.117
5. Gu X, Lin S, Qi K, et al. Application of tungsten oxide and its composites in photocatalysis. Separation and Purification Technology. 2024; 345: 127299. doi: 10.1016/j.seppur.2024.127299 DOI: https://doi.org/10.1016/j.seppur.2024.127299
6. Qureshi F, Yusuf M, Pasha AA, et al. Sustainable and energy efficient hydrogen production via glycerol reforming techniques: A review. International Journal of Hydrogen Energy. 2022; 47(98): 41397-41420. doi: 10.1016/j.ijhydene.2022.04.010 DOI: https://doi.org/10.1016/j.ijhydene.2022.04.010
7. Chen J, Cao J, Zhou J, et al. Mechanism of highly enhanced hydrogen storage by two-dimensional 1T′ MoS2. Physical Chemistry Chemical Physics. 2020; 22(2): 430-436. doi: 10.1039/c9cp04402g DOI: https://doi.org/10.1039/C9CP04402G
8. Qi K, Zhuang C, Zhang M, et al. Sonochemical synthesis of photocatalysts and their applications. Journal of Materials Science & Technology. 2022; 123: 243-256. doi: 10.1016/j.jmst.2022.02.019 DOI: https://doi.org/10.1016/j.jmst.2022.02.019
9. Zhang J, Bifulco A, Amato P, et al. Copper indium sulfide quantum dots in photocatalysis. Journal of Colloid and Interface Science. 2023; 638: 193-219. doi: 10.1016/j.jcis.2023.01.107 DOI: https://doi.org/10.1016/j.jcis.2023.01.107
10. Zhang J, Zhao Y, Qi K, et al. CuInS2 quantum-dot-modified g-C3N4 S-scheme heterojunction photocatalyst for hydrogen production and tetracycline degradation. Journal of Materials Science & Technology. 2024; 172: 145-155. doi: 10.1016/j.jmst.2023.06.042 DOI: https://doi.org/10.1016/j.jmst.2023.06.042
11. Pan J, Shen S, Chen L, et al. Core–Shell Photoanodes for Photoelectrochemical Water Oxidation. Advanced Functional Materials. 2021; 31(36). doi: 10.1002/adfm.202104269 DOI: https://doi.org/10.1002/adfm.202104269
12. Pan J, Wang B, Wang J, et al. Activity and Stability Boosting of an Oxygen‐Vacancy‐Rich BiVO4 Photoanode by NiFe‐MOFs Thin Layer for Water Oxidation. Angewandte Chemie International Edition. 2020; 60(3): 1433-1440. doi: 10.1002/anie.202012550 DOI: https://doi.org/10.1002/anie.202012550
13. Pan J, Wang B, Shen S, et al. Introducing Bidirectional Axial Coordination into BiVO4@Metal Phthalocyanine Core–Shell Photoanodes for Efficient Water Oxidation. Angewandte Chemie International Edition. 2023; 62(38). doi: 10.1002/anie.202307246 DOI: https://doi.org/10.1002/anie.202307246
14. Yang R, Fan Y, Zhang Y, et al. 2D Transition Metal Dichalcogenides for Photocatalysis. Angewandte Chemie International Edition. 2023; 62(13). doi: 10.1002/anie.202218016 DOI: https://doi.org/10.1002/anie.202218016
15. Li Q, Huang L, Dai W, et al. Controlling 1T/2H heterophase junctions in the MoS2 microsphere for the highly efficient photocatalytic hydrogen evolution. Catalysis Science & Technology. 2021; 11(24): 7914-7921. doi: 10.1039/d1cy01340h DOI: https://doi.org/10.1039/D1CY01340H
16. Kuc A, Heine T. The electronic structure calculations of two-dimensional transition-metal dichalcogenides in the presence of external electric and magnetic fields. Chemical Society Reviews. 2015; 44(9): 2603-2614. doi: 10.1039/c4cs00276h DOI: https://doi.org/10.1039/C4CS00276H
17. Li Y, Zhang Y, Tong X, et al. Recent progress on the phase modulation of molybdenum disulphide/diselenide and their applications in electrocatalysis. Journal of Materials Chemistry A. 2021; 9(3): 1418-1428. doi: 10.1039/d0ta08514f DOI: https://doi.org/10.1039/D0TA08514F
18. Fu Q, Han J, Wang X, et al. 2D Transition Metal Dichalcogenides: Design, Modulation, and Challenges in Electrocatalysis. Advanced Materials. 2020; 33(6). doi: 10.1002/adma.201907818 DOI: https://doi.org/10.1002/adma.201907818
19. Wu W, Niu C, Wei C, et al. Activation of MoS2 Basal Planes for Hydrogen Evolution by Zinc. Angewandte Chemie International Edition. 2019; 58(7): 2029-2033. doi: 10.1002/anie.201812475 DOI: https://doi.org/10.1002/anie.201812475
20. Liang Z, Xue Y, Wang X, et al. Co doped MoS2 as cocatalyst considerably improved photocatalytic hydrogen evolution of g-C3N4 in an alkalescent environment. Chemical Engineering Journal. 2021; 421: 130016. doi: 10.1016/j.cej.2021.130016 DOI: https://doi.org/10.1016/j.cej.2021.130016
21. Mohan M, Shetti NP, Aminabhavi TM. Phase dependent performance of MoS2 for supercapacitor applications. Journal of Energy Storage. 2023; 58: 106321. doi: 10.1016/j.est.2022.106321 DOI: https://doi.org/10.1016/j.est.2022.106321
22. Fujishima A, Honda K. Electrochemical Photolysis of Water at a Semiconductor Electrode. Nature. 1972; 238(5358): 37-38. doi: 10.1038/238037a0 DOI: https://doi.org/10.1038/238037a0
23. Chen F, Zhang Y, Huang H. Layered photocatalytic nanomaterials for environmental applications. Chinese Chemical Letters. 2023; 34(3): 107523. doi: 10.1016/j.cclet.2022.05.037 DOI: https://doi.org/10.1016/j.cclet.2022.05.037
24. Cheng XM, Zhao J, Sun WY. Facet-Engineering of Materials for Photocatalytic Application: Status and Future Prospects. EnergyChem. 2022; 4(5): 100084. doi: 10.1016/j.enchem.2022.100084 DOI: https://doi.org/10.1016/j.enchem.2022.100084
25. Ali SA, Ahmad T. Enhanced hydrogen generation via overall water splitting using novel MoS2-BN nanoflowers assembled TiO2 ternary heterostructures. International Journal of Hydrogen Energy. 2023; 48(58): 22044-22059. doi: 10.1016/j.ijhydene.2023.03.118 DOI: https://doi.org/10.1016/j.ijhydene.2023.03.118
26. Ma J, Xu L, Yin Z, et al. “One stone four birds” design atom co-sharing BiOBr/Bi2S3 S-scheme heterojunction photothermal synergistic enhanced full-spectrum photocatalytic activity. Applied Catalysis B: Environmental. 2024; 344: 123601. doi: 10.1016/j.apcatb.2023.123601 DOI: https://doi.org/10.1016/j.apcatb.2023.123601
27. Dong X, Xu L, Ma J, et al. Enhanced interfacial charge transfer and photothermal effect via in-situ construction of atom co-sharing Bi plasmonic/Bi4O5Br2 nanosheet heterojunction towards improved full-spectrum photocatalysis. Chemical Engineering Journal. 2023; 459: 141557. doi: 10.1016/j.cej.2023.141557 DOI: https://doi.org/10.1016/j.cej.2023.141557
28. Li Y, Ma J, Xu L, et al. Enhancement of Charge Separation and NIR Light Harvesting through Construction of 2D–2D Bi4O5I2/BiOBr: Yb3+, Er3+ Z‐Scheme Heterojunctions for Improved Full‐Spectrum Photocatalytic Performance. Advanced Science. 2023; 10(13). doi: 10.1002/advs.202207514 DOI: https://doi.org/10.1002/advs.202207514
29. Kudo A, Miseki Y. Heterogeneous photocatalyst materials for water splitting. Chem Soc Rev. 2009; 38(1): 253-278. doi: 10.1039/b800489g DOI: https://doi.org/10.1039/B800489G
30. Gao W, Zhang S, Wang G, et al. A review on mechanism, applications and influencing factors of carbon quantum dots based photocatalysis. Ceramics International. 2022; 48(24): 35986-35999. doi: 10.1016/j.ceramint.2022.10.116 DOI: https://doi.org/10.1016/j.ceramint.2022.10.116
31. Zhang C, Li Y, Li M, et al. Continuous photocatalysis via photo-charging and dark-discharging for sustainable environmental remediation: Performance, mechanism, and influencing factors. Journal of Hazardous Materials. 2021; 420: 126607. doi: 10.1016/j.jhazmat.2021.126607 DOI: https://doi.org/10.1016/j.jhazmat.2021.126607
32. Hong M, Shi J, Huan Y, et al. Microscopic insights into the catalytic mechanisms of monolayer MoS2 and its heterostructures in hydrogen evolution reaction. Nano Research. 2019; 12(9): 2140-2149. doi: 10.1007/s12274-019-2370-3 DOI: https://doi.org/10.1007/s12274-019-2370-3
33. Li S, Sun J, Guan J. Strategies to improve electrocatalytic and photocatalytic performance of two-dimensional materials for hydrogen evolution reaction. Chinese Journal of Catalysis. 2021; 42: 511-556. doi:10.1016/S1872‐2067(20)63693‐2 DOI: https://doi.org/10.1016/S1872-2067(20)63693-2
34. Zong X, Wu G, Yan H, et al. Photocatalytic H2 Evolution on MoS2/CdS Catalysts under Visible Light Irradiation. The Journal of Physical Chemistry C. 2010; 114(4): 1963-1968. doi: 10.1021/jp904350e DOI: https://doi.org/10.1021/jp904350e
35. Chen J, Wu X, Yin L, et al. One‐pot Synthesis of CdS Nanocrystals Hybridized with Single‐Layer Transition‐Metal Dichalcogenide Nanosheets for Efficient Photocatalytic Hydrogen Evolution. Angewandte Chemie International Edition. 2014; 54(4): 1210-1214. doi: 10.1002/anie.201410172 DOI: https://doi.org/10.1002/anie.201410172
36. Kang Y, Gong Y, Hu Z, et al. Plasmonic hot electron enhanced MoS2 photocatalysis in hydrogen evolution. Nanoscale. 2015; 7(10): 4482-4488. doi: 10.1039/c4nr07303g DOI: https://doi.org/10.1039/C4NR07303G
37. Liu C, Wang L, Tang Y, et al. Vertical single or few-layer MoS2 nanosheets rooting into TiO2 nanofibers for highly efficient photocatalytic hydrogen evolution. Applied Catalysis B: Environmental. 2015; 164: 1-9. doi: 10.1016/j.apcatb.2014.08.046 DOI: https://doi.org/10.1016/j.apcatb.2014.08.046
38. Xue Y, Min S, Meng J, et al. Light-induced confined growth of amorphous Co doped MoSx nanodots on TiO2 nanoparticles for efficient and stable in situ photocatalytic H2 evolution. International Journal of Hydrogen Energy. 2019; 44(16): 8133-8143. doi: 10.1016/j.ijhydene.2019.02.057 DOI: https://doi.org/10.1016/j.ijhydene.2019.02.057
39. Alharthi FA, Hasan I. Visible light-driven efficient photocatalytic hydrogen production using nickel-doped molybdenum disulfide (Ni@MoS2) nanoflowers. Journal of Materials Science. 2024; 59(8): 3394-3405. doi: 10.1007/s10853-024-09435-5 DOI: https://doi.org/10.1007/s10853-024-09435-5
40. Sorgenfrei NLAN, Giangrisostomi E, Jay RM, et al. Photodriven Transient Picosecond Top‐Layer Semiconductor to Metal Phase‐Transition in p‐Doped Molybdenum Disulfide. Advanced Materials. 2021; 33(14). doi: 10.1002/adma.202006957 DOI: https://doi.org/10.1002/adma.202006957
41. Xin X, Song Y, Guo S, et al. One-step synthesis of P-doped MoS2 for efficient photocatalytic hydrogen production. Journal of Alloys and Compounds. 2020; 829: 154635. doi: 10.1016/j.jallcom.2020.154635 DOI: https://doi.org/10.1016/j.jallcom.2020.154635
42. Xu ML, Tang X, Wang Y, et al. Phosphorus-doped molybdenum disulfide facilitating the photocatalytic hydrogen production activity of CdS nanorod. New Journal of Chemistry. 2019; 43(14): 5335-5340. doi: 10.1039/c9nj00411d DOI: https://doi.org/10.1039/C9NJ00411D
43. Wang L, Xie L, Zhao W, et al. Oxygen-facilitated dynamic active-site generation on strained MoS2 during photo-catalytic hydrogen evolution. Chemical Engineering Journal. 2021; 405: 127028. doi: 10.1016/j.cej.2020.127028 DOI: https://doi.org/10.1016/j.cej.2020.127028
44. Jaramillo TF, Jørgensen KP, Bonde J, et al. Identification of Active Edge Sites for Electrochemical H2 Evolution from MoS2 Nanocatalysts. Science. 2007; 317(5834): 100-102. doi: 10.1126/science.1141483 DOI: https://doi.org/10.1126/science.1141483
45. Hinnemann B, Moses PG, Bonde J, et al. Biomimetic Hydrogen Evolution: MoS2 Nanoparticles as Catalyst for Hydrogen Evolution. Journal of the American Chemical Society. 2005; 127(15): 5308-5309. doi: 10.1021/ja0504690 DOI: https://doi.org/10.1021/ja0504690
46. Li Y, Wu S, Zheng J, et al. 2D photocatalysts with tuneable supports for enhanced photocatalytic water splitting. Materials Today. 2020; 41: 34-43. doi: 10.1016/j.mattod.2020.05.018 DOI: https://doi.org/10.1016/j.mattod.2020.05.018
47. Zhou X, Hao H, Zhang YJ, et al. Patterning of transition metal dichalcogenides catalyzed by surface plasmons with atomic precision. Chem. 2021; 7(6): 1626-1638. doi: 10.1016/j.chempr.2021.03.011 DOI: https://doi.org/10.1016/j.chempr.2021.03.011
48. Liu J, Liu H, Peng W, et al. High-yield exfoliation of MoS2 (WS2) monolayers towards efficient photocatalytic hydrogen evolution. Chemical Engineering Journal. 2022; 431: 133286. doi: 10.1016/j.cej.2021.133286 DOI: https://doi.org/10.1016/j.cej.2021.133286
49. Li X, Sun X, Yu H, et al. Pseudo metallic (1T) molybdenum disulfide for efficient photo/electrocatalytic water splitting. Applied Catalysis B: Environmental. 2022; 307: 121156. doi: 10.1016/j.apcatb.2022.121156 DOI: https://doi.org/10.1016/j.apcatb.2022.121156
50. Hu X, Jin J, Wang Y, et al. Au/MoS2 tips as auxiliary rate aligners for the photocatalytic generation of syngas with a tunable composition. Applied Catalysis B: Environmental. 2022; 308: 121219. doi: 10.1016/j.apcatb.2022.121219 DOI: https://doi.org/10.1016/j.apcatb.2022.121219
51. Parzinger E, Miller B, Blaschke B, et al. Photocatalytic Stability of Single- and Few-Layer MoS2. ACS Nano. 2015; 9(11): 11302-11309. doi: 10.1021/acsnano.5b04979 DOI: https://doi.org/10.1021/acsnano.5b04979
52. Peng R, Ma X, Hood ZD, et al. Synergizing plasmonic Au nanocages with 2D MoS2 nanosheets for significant enhancement in photocatalytic hydrogen evolution. Journal of Materials Chemistry A. 2023; 11(31): 16714-16723. doi: 10.1039/d3ta01657a DOI: https://doi.org/10.1039/D3TA01657A
53. Zhao X, Chen S, Yin H, et al. Perovskite Microcrystals with Intercalated Monolayer MoS2 Nanosheets as Advanced Photocatalyst for Solar-Powered Hydrogen Generation. Matter. 2020; 3(3): 935-949. doi: 10.1016/j.matt.2020.07.004 DOI: https://doi.org/10.1016/j.matt.2020.07.004
54. Guan W, Li Y, Zhong Q, et al. Fabricating MAPbI3/MoS2 Composites for Improved Photocatalytic Performance. Nano Letters. 2020; 21(1): 597-604. doi: 10.1021/acs.nanolett.0c04073 DOI: https://doi.org/10.1021/acs.nanolett.0c04073
55. Khalid NR, Israr Z, Tahir MB, et al. Highly efficient Bi2O3/MoS2 p-n heterojunction photocatalyst for H2 evolution from water splitting. International Journal of Hydrogen Energy. 2020; 45(15): 8479-8489. doi: 10.1016/j.ijhydene.2020.01.031 DOI: https://doi.org/10.1016/j.ijhydene.2020.01.031
56. Song T, Wang J, Su L, et al. Promotion effect of rhenium on MoS2/ReS2@CdS nanostructures for photocatalytic hydrogen production. Molecular Catalysis. 2021; 516: 111939. doi: 10.1016/j.mcat.2021.111939 DOI: https://doi.org/10.1016/j.mcat.2021.111939
57. Zhang J, Xing C, Shi F. MoS2/Ti3C2 heterostructure for efficient visible-light photocatalytic hydrogen generation. International Journal of Hydrogen Energy. 2020; 45(11): 6291-6301. doi: 10.1016/j.ijhydene.2019.12.109 DOI: https://doi.org/10.1016/j.ijhydene.2019.12.109
58. Rothfuss ARM, Ayala JR, Handy JV, et al. Linker-Assisted Assembly of Ligand-Bridged CdS/MoS2 Heterostructures: Tunable Light-Harvesting Properties and Ligand-Dependent Control of Charge-Transfer Dynamics and Photocatalytic Hydrogen Evolution. ACS Applied Materials & Interfaces. 2023; 15(33): 39966-39979. doi: 10.1021/acsami.3c06722 DOI: https://doi.org/10.1021/acsami.3c06722
59. Ou‐Yang H, Xu H, Zhang X, et al. Selective‐Epitaxial Hybrid of Tripartite Semiconducting Sulfides for Enhanced Solar‐to‐Hydrogen Conversion. Small. 2022; 18(38). doi: 10.1002/smll.202202109 DOI: https://doi.org/10.1002/smll.202202109
60. Yanalak G, Koç BK, Yılmaz S, et al. The effect of Ni and Co co-catalysts on the catalytic activity of mesoporous graphitic carbon nitride/black phosphorus/molybdenum disulfide heterojunctions in solar-driven hydrogen evolution. Journal of Environmental Chemical Engineering. 2023; 11(5): 111084. doi: 10.1016/j.jece.2023.111084 DOI: https://doi.org/10.1016/j.jece.2023.111084
61. Peng R, Liang L, Hood ZD, et al. In-Plane Heterojunctions Enable Multiphasic Two-Dimensional (2D) MoS2 Nanosheets As Efficient Photocatalysts for Hydrogen Evolution from Water Reduction. ACS Catalysis. 2016; 6(10): 6723-6729. doi: 10.1021/acscatal.6b02076 DOI: https://doi.org/10.1021/acscatal.6b02076
62. Das S, Sharma U, Mukherjee B, et al. Polygonal gold nanocrystal induced efficient phase transition in 2D-MoS2 for enhancing photo-electrocatalytic hydrogen generation. Nanotechnology. 2023; 34(14): 145202. doi: 10.1088/1361-6528/acade6 DOI: https://doi.org/10.1088/1361-6528/acade6
63. Xin X, Song Y, Guo S, et al. In-situ growth of high-content 1T phase MoS2 confined in the CuS nanoframe for efficient photocatalytic hydrogen evolution. Applied Catalysis B: Environmental. 2020; 269: 118773. doi: 10.1016/j.apcatb.2020.118773 DOI: https://doi.org/10.1016/j.apcatb.2020.118773
64. Liang Z, Xue Y, Wang X, et al. Structure engineering of 1T/2H multiphase MoS2 via oxygen incorporation over 2D layered porous g-C3N4 for remarkably enhanced photocatalytic hydrogen evolution. Materials Today Nano. 2022; 18: 100204. doi: 10.1016/j.mtnano.2022.100204 DOI: https://doi.org/10.1016/j.mtnano.2022.100204
65. Zhao Y, Gao J, Bian X, et al. From the perspective of experimental practice: High-throughput computational screening in photocatalysis. Green Energy & Environment. 2024; 9(1): 1-6. doi: 10.1016/j.gee.2023.05.008 DOI: https://doi.org/10.1016/j.gee.2023.05.008
66. Xu C, Zhou G, Alexeev EM, et al. Ultrafast Electronic Relaxation Dynamics of Atomically Thin MoS2 Is Accelerated by Wrinkling. ACS Nano. 2023; 17(17): 16682-16694. doi: 10.1021/acsnano.3c02917 DOI: https://doi.org/10.1021/acsnano.3c02917
67. Zhou P, Navid IA, Ma Y, et al. Solar-to-hydrogen efficiency of more than 9% in photocatalytic water splitting. Nature. 2023; 613(7942): 66-70. doi: 10.1038/s41586-022-05399-1 DOI: https://doi.org/10.1038/s41586-022-05399-1
68. Zhao Y, Ding C, Zhu J, et al. A Hydrogen Farm Strategy for Scalable Solar Hydrogen Production with Particulate Photocatalysts. Angewandte Chemie International Edition. 2020; 59(24): 9653-9658. doi: 10.1002/anie.202001438 DOI: https://doi.org/10.1002/anie.202001438
69. Zhai W, Ma Y, Chen D, et al. Recent progress on the long‐term stability of hydrogen evolution reaction electrocatalysts. InfoMat. 2022; 4(9). doi: 10.1002/inf2.12357 DOI: https://doi.org/10.1002/inf2.12357
70. Diyali S, Diyali N, Biswas B. Coordination-driven electrocatalysts as an evolving wave of enthusiasm for sustainable hydrogen production. Coordination Chemistry Reviews. 2024; 500: 215496. doi: 10.1016/j.ccr.2023.215496 DOI: https://doi.org/10.1016/j.ccr.2023.215496
71. Zhang J, Wu J, Guo H, et al. Unveiling Active Sites for the Hydrogen Evolution Reaction on Monolayer MoS2. Advanced Materials. 2017; 29(42). doi: 10.1002/adma.201701955 DOI: https://doi.org/10.1002/adma.201701955
72. Chen J, Liu G, Zhu Y, et al. Ag@MoS2 Core–Shell Heterostructure as SERS Platform to Reveal the Hydrogen Evolution Active Sites of Single-Layer MoS2. Journal of the American Chemical Society. 2020; 142(15): 7161-7167. doi: 10.1021/jacs.0c01649 DOI: https://doi.org/10.1021/jacs.0c01649
73. Ekspong J, Sharifi T, Shchukarev A, et al. Stabilizing Active Edge Sites in Semicrystalline Molybdenum Sulfide by Anchorage on Nitrogen‐Doped Carbon Nanotubes for Hydrogen Evolution Reaction. Advanced Functional Materials. 2016; 26(37): 6766-6776. doi: 10.1002/adfm.201601994 DOI: https://doi.org/10.1002/adfm.201601994
74. Li H, Tsai C, Koh AL, et al. Correction: Corrigendum: Activating and optimizing MoS2 basal planes for hydrogen evolution through the formation of strained sulphur vacancies. Nature Materials. 2016; 15(3): 364-364. doi: 10.1038/nmat4564 DOI: https://doi.org/10.1038/nmat4564
75. Kim Y, Jackson DHK, Lee D, et al. In Situ Electrochemical Activation of Atomic Layer Deposition Coated MoS2 Basal Planes for Efficient Hydrogen Evolution Reaction. Advanced Functional Materials. 2017; 27(34). doi: 10.1002/adfm.201701825 DOI: https://doi.org/10.1002/adfm.201701825
76. Kibsgaard J, Chen Z, Reinecke BN, et al. Engineering the surface structure of MoS2 to preferentially expose active edge sites for electrocatalysis. Nature Materials. 2012; 11(11): 963-969. doi: 10.1038/nmat3439 DOI: https://doi.org/10.1038/nmat3439
77. Tsai C, Li H, Park S, et al. Electrochemical generation of sulfur vacancies in the basal plane of MoS2 for hydrogen evolution. Nature Communications. 2017; 8(1). doi: 10.1038/ncomms15113 DOI: https://doi.org/10.1038/ncomms15113
78. Liu X, Jiang X, Shao G, et al. Activating the Electrocatalysis of MoS2 Basal Plane for Hydrogen Evolution via Atomic Defect Configurations. Small. 2022; 18(22). doi: 10.1002/smll.202200601 DOI: https://doi.org/10.1002/smll.202200601
79. Man P, Jiang S, Leung KH, et al. Salt‐Induced High‐Density Vacancy‐Rich 2D MoS2 for Efficient Hydrogen Evolution. Advanced Materials. 2023; 36(17). doi: 10.1002/adma.202304808 DOI: https://doi.org/10.1002/adma.202304808
80. Xiao W, Liu P, Zhang J, et al. Dual‐Functional N Dopants in Edges and Basal Plane of MoS2 Nanosheets Toward Efficient and Durable Hydrogen Evolution. Advanced Energy Materials. 2016; 7(7). doi: 10.1002/aenm.201602086 DOI: https://doi.org/10.1002/aenm.201602086
81. Liu P, Zhu J, Zhang J, et al. P Dopants Triggered New Basal Plane Active Sites and Enlarged Interlayer Spacing in MoS2 Nanosheets toward Electrocatalytic Hydrogen Evolution. ACS Energy Letters. 2017; 2(4): 745-752. doi: 10.1021/acsenergylett.7b00111 DOI: https://doi.org/10.1021/acsenergylett.7b00111
82. Yang YQ, Zhao CX, Bai SY, et al. Activating MoS2 basal planes for hydrogen evolution through the As doping and strain. Physics Letters A. 2019; 383(24): 2997-3000. doi: 10.1016/j.physleta.2019.06.036 DOI: https://doi.org/10.1016/j.physleta.2019.06.036
83. Anjum MAR, Jeong HY, Lee MH, et al. Efficient Hydrogen Evolution Reaction Catalysis in Alkaline Media by All‐in‐One MoS2 with Multifunctional Active Sites. Advanced Materials. 2018; 30(20). doi: 10.1002/adma.201707105 DOI: https://doi.org/10.1002/adma.201707105
84. Lukowski MA, Daniel AS, Meng F, et al. Enhanced Hydrogen Evolution Catalysis from Chemically Exfoliated Metallic MoS2 Nanosheets. Journal of the American Chemical Society. 2013; 135(28): 10274-10277. doi: 10.1021/ja404523s DOI: https://doi.org/10.1021/ja404523s
85. Yin Y, Han J, Zhang Y, et al. Contributions of Phase, Sulfur Vacancies, and Edges to the Hydrogen Evolution Reaction Catalytic Activity of Porous Molybdenum Disulfide Nanosheets. Journal of the American Chemical Society. 2016; 138(25): 7965-7972. doi: 10.1021/jacs.6b03714 DOI: https://doi.org/10.1021/jacs.6b03714
86. Chang K, Hai X, Pang H, et al. Targeted Synthesis of 2H‐ and 1T‐Phase MoS2 Monolayers for Catalytic Hydrogen Evolution. Advanced Materials. 2016; 28(45): 10033-10041. doi: 10.1002/adma.201603765 DOI: https://doi.org/10.1002/adma.201603765
87. Zhu J, Wang Z, Yu H, et al. Argon Plasma Induced Phase Transition in Monolayer MoS2. Journal of the American Chemical Society. 2017; 139(30): 10216-10219. doi: 10.1021/jacs.7b05765 DOI: https://doi.org/10.1021/jacs.7b05765
88. Liu Z, Zhao L, Liu Y, et al. Vertical nanosheet array of 1T phase MoS2 for efficient and stable hydrogen evolution. Applied Catalysis B: Environmental. 2019; 246: 296-302. doi: 10.1016/j.apcatb.2019.01.062 DOI: https://doi.org/10.1016/j.apcatb.2019.01.062
89. Li J, Listwan A, Liang J, et al. High proportion of 1 T phase MoS2 prepared by a simple solvothermal method for high-efficiency electrocatalytic hydrogen evolution. Chemical Engineering Journal. 2021; 422: 130100. doi: 10.1016/j.cej.2021.130100 DOI: https://doi.org/10.1016/j.cej.2021.130100
90. Yang J, Xu Q, Zheng Y, et al. Phase Engineering of Metastable Transition Metal Dichalcogenides via Ionic Liquid Assisted Synthesis. ACS Nano. 2022; 16(9): 15215-15225. doi: 10.1021/acsnano.2c06549 DOI: https://doi.org/10.1021/acsnano.2c06549
91. Ekspong J, Sandström R, Rajukumar LP, et al. Stable Sulfur‐Intercalated 1T′ MoS2 on Graphitic Nanoribbons as Hydrogen Evolution Electrocatalyst. Advanced Functional Materials. 2018; 28(46). doi: 10.1002/adfm.201802744 DOI: https://doi.org/10.1002/adfm.201802744
92. Gao B, Zhao Y, Du X, et al. Electron injection induced phase transition of 2H to 1T MoS2 by cobalt and nickel substitutional doping. Chemical Engineering Journal. 2021; 411: 128567. doi: 10.1016/j.cej.2021.128567 DOI: https://doi.org/10.1016/j.cej.2021.128567
93. Jiang L, Zhang YJ, Luo XH, et al. Se and O co-insertion induce the transition of MoS2 from 2H to 1T phase for designing high-active electrocatalyst of hydrogen evolution reaction. Chemical Engineering Journal. 2021; 425: 130611. doi: 10.1016/j.cej.2021.130611 DOI: https://doi.org/10.1016/j.cej.2021.130611
94. Guo X, Song E, Zhao W, et al. Charge self-regulation in 1T’’’-MoS2 structure with rich S vacancies for enhanced hydrogen evolution activity. Nature Communications. 2022; 13(1). doi: 10.1038/s41467-022-33636-8 DOI: https://doi.org/10.1038/s41467-022-33636-8
95. Yang Y, Zhang K, Lin H, et al. MoS2–Ni3S2 Heteronanorods as Efficient and Stable Bifunctional Electrocatalysts for Overall Water Splitting. ACS Catalysis. 2017; 7(4): 2357-2366. doi: 10.1021/acscatal.6b03192 DOI: https://doi.org/10.1021/acscatal.6b03192
96. Kim M, Anjum MAR, Lee M, et al. Activating MoS2 Basal Plane with Ni2P Nanoparticles for Pt‐Like Hydrogen Evolution Reaction in Acidic Media. Advanced Functional Materials. 2019; 29(10). doi: 10.1002/adfm.201809151 DOI: https://doi.org/10.1002/adfm.201809151
97. Kim M, Anjum MAR, Choi M, et al. Covalent 0D–2D Heterostructuring of Co9S8–MoS2 for Enhanced Hydrogen Evolution in All pH Electrolytes. Advanced Functional Materials. 2020; 30(40). doi: 10.1002/adfm.202002536 DOI: https://doi.org/10.1002/adfm.202002536
98. Wang S, Zhang D, Li B, et al. Ultrastable In‐Plane 1T–2H MoS2 Heterostructures for Enhanced Hydrogen Evolution Reaction. Advanced Energy Materials. 2018; 8(25). doi: 10.1002/aenm.201801345 DOI: https://doi.org/10.1002/aenm.201801345
99. Cheng Z, Xiao Y, Wu W, et al. All-pH-Tolerant In-Plane Heterostructures for Efficient Hydrogen Evolution Reaction. ACS Nano. 2021; 15(7): 11417-11427. doi: 10.1021/acsnano.1c01024 DOI: https://doi.org/10.1021/acsnano.1c01024
100. Sun Z, Lin L, Yuan M, et al. Mott–Schottky heterostructure induce the interfacial electron redistribution of MoS2 for boosting pH-universal hydrogen evolution with Pt-like activity. Nano Energy. 2022; 101: 107563. doi: 10.1016/j.nanoen.2022.107563 DOI: https://doi.org/10.1016/j.nanoen.2022.107563
101. Li L, Qin Z, Ries L, et al. Role of Sulfur Vacancies and Undercoordinated Mo Regions in MoS2 Nanosheets toward the Evolution of Hydrogen. ACS Nano. 2019; 13(6): 6824-6834. doi: 10.1021/acsnano.9b01583 DOI: https://doi.org/10.1021/acsnano.9b01583
102. Zhang W, Liao X, Pan X, et al. Superior Hydrogen Evolution Reaction Performance in 2H‐MoS2 to that of 1T Phase. Small. 2019; 15(31). doi: 10.1002/smll.201900964 DOI: https://doi.org/10.1002/smll.201900964
103. Hou X, Zhou H, Zhao M, et al. MoS2 Nanoplates Embedded in Co–N-Doped Carbon Nanocages as Efficient Catalyst for HER and OER. ACS Sustainable Chemistry & Engineering. 2020; 8(14): 5724-5733. doi: 10.1021/acssuschemeng.0c00810 DOI: https://doi.org/10.1021/acssuschemeng.0c00810
104. Wang X, Zhang Y, Si H, et al. Single-Atom Vacancy Defect to Trigger High-Efficiency Hydrogen Evolution of MoS2. Journal of the American Chemical Society. 2020; 142(9): 4298-4308. doi: 10.1021/jacs.9b12113 DOI: https://doi.org/10.1021/jacs.9b12113
105. Ye G, Gong Y, Lin J, et al. Defects Engineered Monolayer MoS2 for Improved Hydrogen Evolution Reaction. Nano Letters. 2016; 16(2): 1097-1103. doi: 10.1021/acs.nanolett.5b04331 DOI: https://doi.org/10.1021/acs.nanolett.5b04331
106. Zhan W, Zhai X, Li Y, et al. Regulating Local Atomic Environment around Vacancies for Efficient Hydrogen Evolution. ACS Nano. 2024; 18(14): 10312-10323. doi: 10.1021/acsnano.4c02283 DOI: https://doi.org/10.1021/acsnano.4c02283
107. Li Y, Zhang R, Zhou W, et al. Hierarchical MoS2 Hollow Architectures with Abundant Mo Vacancies for Efficient Sodium Storage. ACS Nano. 2019; 13(5): 5533-5540. doi: 10.1021/acsnano.9b00383 DOI: https://doi.org/10.1021/acsnano.9b00383
108. Ge J, Chen Y, Zhao Y, et al. Activated MoS2 by Constructing Single Atomic Cation Vacancies for Accelerated Hydrogen Evolution Reaction. ACS Applied Materials & Interfaces. 2022; 14(23): 26846-26857. doi: 10.1021/acsami.2c06708 DOI: https://doi.org/10.1021/acsami.2c06708
109. Shi Y, Zhou Y, Yang DR, et al. Energy Level Engineering of MoS2 by Transition-Metal Doping for Accelerating Hydrogen Evolution Reaction. Journal of the American Chemical Society. 2017; 139(43): 15479-15485. doi: 10.1021/jacs.7b08881 DOI: https://doi.org/10.1021/jacs.7b08881
110. Xu J, Shao G, Tang X, et al. Frenkel-defected monolayer MoS2 catalysts for efficient hydrogen evolution. Nature Communications. 2022; 13(1). doi: 10.1038/s41467-022-29929-7 DOI: https://doi.org/10.1038/s41467-022-29929-7
111. Xiong Q, Wang Y, Liu P, et al. Cobalt Covalent Doping in MoS2 to Induce Bifunctionality of Overall Water Splitting. Advanced Materials. 2018; 30(29). doi: 10.1002/adma.201801450 DOI: https://doi.org/10.1002/adma.201801450
112. Xue Y, Bai X, Xu Y, et al. Vertically oriented Ni-doped MoS2 nanosheets supported on hollow carbon microtubes for enhanced hydrogen evolution reaction and water splitting. Composites Part B: Engineering. 2021; 224: 109229. doi: 10.1016/j.compositesb.2021.109229 DOI: https://doi.org/10.1016/j.compositesb.2021.109229
113. Li C, Zhu L, Wu Z, et al. Phase Engineering of W‐Doped MoS2 by Magneto‐Hydrothermal Synthesis for Hydrogen Evolution Reaction. Small. 2023; 19(48). doi: 10.1002/smll.202303646 DOI: https://doi.org/10.1002/smll.202303646
114. Kong L, Gao C, Liu Z, et al. Cerium-doped 1 T phase enriched MoS2 flower-like nanoflakes for boosting hydrogen evolution reaction. Chemical Engineering Journal. 2024; 479: 147725. doi: 10.1016/j.cej.2023.147725 DOI: https://doi.org/10.1016/j.cej.2023.147725
115. Li R, Yang L, Xiong T, et al. Nitrogen doped MoS2 nanosheets synthesized via a low-temperature process as electrocatalysts with enhanced activity for hydrogen evolution reaction. Journal of Power Sources. 2017; 356: 133-139. doi: 10.1016/j.jpowsour.2017.04.060 DOI: https://doi.org/10.1016/j.jpowsour.2017.04.060
116. Zhang P, Xu B, Chen G, et al. Large-scale synthesis of nitrogen doped MoS2 quantum dots for efficient hydrogen evolution reaction. Electrochimica Acta. 2018; 270: 256-263. doi: 10.1016/j.electacta.2018.03.097 DOI: https://doi.org/10.1016/j.electacta.2018.03.097
117. Ge J, Zhang D, Jin J, et al. Oxygen atoms substituting sulfur atoms of MoS2 to activate the basal plane and induce the phase transition for boosting hydrogen evolution. Materials Today Energy. 2021; 22: 100854. doi: 10.1016/j.mtener.2021.100854 DOI: https://doi.org/10.1016/j.mtener.2021.100854
118. Zhang R, Zhang M, Yang H, et al. Creating Fluorine‐Doped MoS2 Edge Electrodes with Enhanced Hydrogen Evolution Activity. Small Methods. 2021; 5(11). doi: 10.1002/smtd.202100612 DOI: https://doi.org/10.1002/smtd.202100612
119. Shi Y, Zhang D, Miao H, et al. Amorphous/2H-MoS2 nanoflowers with P doping and S vacancies to achieve efficient pH-universal hydrogen evolution at high current density. Science China Chemistry. 2022; 65(9): 1829-1837. doi: 10.1007/s11426-022-1287-4 DOI: https://doi.org/10.1007/s11426-022-1287-4
120. Li F, Li J, Cao Z, et al. MoS2 quantum dot decorated RGO: a designed electrocatalyst with high active site density for the hydrogen evolution reaction. Journal of Materials Chemistry A. 2015; 3(43): 21772-21778. doi: 10.1039/c5ta05219j DOI: https://doi.org/10.1039/C5TA05219J
121. Biroju RK, Das D, Sharma R, et al. Hydrogen Evolution Reaction Activity of Graphene–MoS2 van der Waals Heterostructures. ACS Energy Letters. 2017; 2(6): 1355-1361. doi: 10.1021/acsenergylett.7b00349 DOI: https://doi.org/10.1021/acsenergylett.7b00349
122. Zhang CL, Xie Y, Liu JT, et al. 1D Core−Shell MOFs derived CoP Nanoparticles-Embedded N-doped porous carbon nanotubes anchored with MoS2 nanosheets as efficient bifunctional electrocatalysts. Chemical Engineering Journal. 2021; 419: 129977. doi: 10.1016/j.cej.2021.129977 DOI: https://doi.org/10.1016/j.cej.2021.129977
123. Zhang X, Yang P, Jiang SP. Ni diffusion in vertical growth of MoS2 nanosheets on carbon nanotubes towards highly efficient hydrogen evolution. Carbon. 2021; 175: 176-186. doi: 10.1016/j.carbon.2021.01.010 DOI: https://doi.org/10.1016/j.carbon.2021.01.010
124. Yu H, Xue Y, Hui L, et al. Controlled Growth of MoS2 Nanosheets on 2D N‐Doped Graphdiyne Nanolayers for Highly Associated Effects on Water Reduction. Advanced Functional Materials. 2018; 28(19). doi: 10.1002/adfm.201707564 DOI: https://doi.org/10.1002/adfm.201707564
125. Hui L, Xue Y, He F, et al. Efficient hydrogen generation on graphdiyne-based heterostructure. Nano Energy. 2019; 55: 135-142. doi: 10.1016/j.nanoen.2018.10.062 DOI: https://doi.org/10.1016/j.nanoen.2018.10.062
126. Song L, Zhang X, Zhu S, et al. Hydrogen spillover effect enhanced by carbon quantum dots activated MoS2. Carbon. 2022; 199: 63-69. doi: 10.1016/j.carbon.2022.07.071 DOI: https://doi.org/10.1016/j.carbon.2022.07.071
127. Attanayake NH, Abeyweera SC, Thenuwara AC, et al. Vertically aligned MoS2 on Ti3C2 (MXene) as an improved HER catalyst. Journal of Materials Chemistry A. 2018; 6(35): 16882-16889. doi: 10.1039/c8ta05033c DOI: https://doi.org/10.1039/C8TA05033C
128. Wu X, Wang Z, Yu M, et al. Stabilizing the MXenes by Carbon Nanoplating for Developing Hierarchical Nanohybrids with Efficient Lithium Storage and Hydrogen Evolution Capability. Advanced Materials. 2017; 29(24). doi: 10.1002/adma.201607017 DOI: https://doi.org/10.1002/adma.201607017
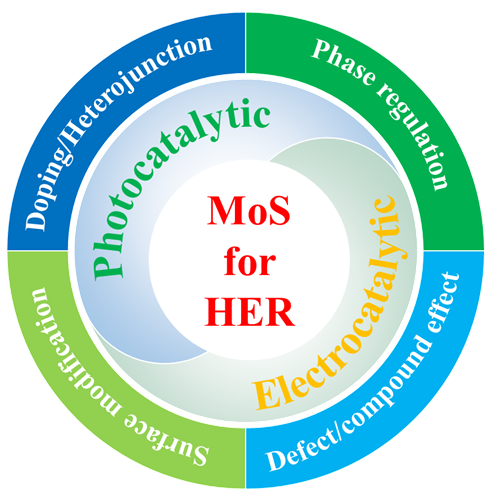
Downloads
Published
How to Cite
Issue
Section
License
Copyright (c) 2024 Liang Xu, Zhiguo Song, Hongkun Chen, Yongjin Li, Jingwei Li, Ruchun Li

This work is licensed under a Creative Commons Attribution 4.0 International License.