A review of cold plasma for catalyst synthesis and modification
DOI:
https://doi.org/10.18686/cest.v2i1.131Keywords:
cold plasma; catalysts synthesis; surface modification; plasma dispersionAbstract
Cold plasma has been extensively studied and developed in the field of energy storage and conversion, with a focus on its ability to assist in catalyst synthesis, surface modification, the introduction of heteroatoms, the generation of defects and vacancies, the improvement of catalyst dispersion, and the reduction of particle size. In contrast to conventional calcination and chemical methods, the energy from cold plasma can be transferred directly to the catalyst and carrier during the treatment process, which can improve the interaction between the loaded catalyst and carrier by changing the internal structure and surface morphology of the catalyst. Therefore, these properties make cold plasma quite green, safe, and efficient for catalyst synthesis and modification. In this paper, the characteristics and applications of various cold plasma technologies, as well as the synergistic treatment of cold plasma technology with thermodynamic principles on catalysts, are analyzed. Based on current research progress, this paper provides a summary and outlook on the synthesis and modification of catalysts using cold plasma.
References
Adamovich I, Baalrud SD, Bogaerts A, et al. The 2017 Plasma Roadmap: Low temperature plasma science and technology. Journal of Physics D: Applied Physics. 2017; 50(32): 323001. doi: 10.1088/1361-6463/aa76f5 DOI: https://doi.org/10.1088/1361-6463/aa76f5
Lee CGN, Kanarik KJ, Gottscho RA. The grand challenges of plasma etching: a manufacturing perspective. Journal of Physics D: Applied Physics. 2014; 47(27): 273001. doi: 10.1088/0022-3727/47/27/273001 DOI: https://doi.org/10.1088/0022-3727/47/27/273001
Bogaerts A, Neyts E, Gijbels R, 2002. Gas Discharge Plasmas and their Applications. Spectrochimica Acta Part B. 57: 609-658. doi: 10.1016/S0584-8547(01)00406-2 DOI: https://doi.org/10.1016/S0584-8547(01)00406-2
Zhang B, Shang X, Jiang Z, et al. Atmospheric-Pressure Plasma Jet-Induced Ultrafast Construction of an Ultrathin Nonstoichiometric Nickel Oxide Layer with Mixed Ni3+/Ni2+ Ions and Rich Oxygen Defects as an Efficient Electrocatalyst for Oxygen Evolution Reaction. ACS Applied Energy Materials. 2021; 4(5): 5059-5069. doi: 10.1021/acsaem.1c00623 DOI: https://doi.org/10.1021/acsaem.1c00623
Witvrouwen T, Paulussen S, Sels B. The Use of Non‐Equilibrium Plasmas for the Synthesis of Heterogeneous Catalysts. Plasma Processes and Polymers. 2012; 9(8): 750-760. doi: 10.1002/ppap.201200004 DOI: https://doi.org/10.1002/ppap.201200004
Snoeckx R, Bogaerts A. Plasma technology – a novel solution for CO2 conversion? Chemical Society Reviews. 2017; 46(19): 5805-5863. doi: 10.1039/c6cs00066e DOI: https://doi.org/10.1039/C6CS00066E
Whitehead JC. Plasma-catalysis: Is it just a question of scale? Frontiers of Chemical Science and Engineering. 2019; 13(2): 264-273. doi: 10.1007/s11705-019-1794-3 DOI: https://doi.org/10.1007/s11705-019-1794-3
Neyts EC. PECVD growth of carbon nanotubes: From experiment to simulation. Journal of Vacuum Science & Technology B, Nanotechnology and Microelectronics: Materials, Processing, Measurement, and Phenomena. 2012; 30(3). doi: 10.1116/1.3702806 DOI: https://doi.org/10.1116/1.3702806
Kato T, Hatakeyama R. Direct Growth of Doping-Density-Controlled Hexagonal Graphene on SiO2 Substrate by Rapid-Heating Plasma CVD. ACS Nano. 2012; 6(10): 8508-8515. doi: 10.1021/nn302290z DOI: https://doi.org/10.1021/nn302290z
Piferi C, Riccardi C. A study on propane depletion by surface dielectric barrier discharges. Cleaner Engineering and Technology. 2022; 8: 100486. doi: 10.1016/j.clet.2022.100486 DOI: https://doi.org/10.1016/j.clet.2022.100486
Kato R, Tsugawa K, Okigawa Y, et al. Bilayer graphene synthesis by plasma treatment of copper foils without using a carbon-containing gas. Carbon. 2014; 77: 823-828. doi: 10.1016/j.carbon.2014.05.087 DOI: https://doi.org/10.1016/j.carbon.2014.05.087
Purushothaman V, Jeganathan K. Investigations on the role of Ni-catalyst for the VLS growth of quasi-aligned GaN nanowires by chemical vapor deposition. Journal of Nanoparticle Research. 2013; 15(7). doi: 10.1007/s11051-013-1789-9 DOI: https://doi.org/10.1007/s11051-013-1789-9
Schmidt M, Kettlitz M, Kolb JF. How activated carbon improves the performance of non-thermal plasma removing methyl ethyl ketone from a gas stream. Cleaner Engineering and Technology. 2021; 4: 100234. doi: 10.1016/j.clet.2021.100234 DOI: https://doi.org/10.1016/j.clet.2021.100234
Tang WC, Hong FCN. Growths of indium gallium nitride nanowires by plasma-assisted chemical vapor deposition. Thin Solid Films. 2014; 570: 315-320. doi: 10.1016/j.tsf.2014.04.053 DOI: https://doi.org/10.1016/j.tsf.2014.04.053
Harling AM, Demidyuk V, Fischer SJ, et al. Plasma-catalysis destruction of aromatics for environmental clean-up: Effect of temperature and configuration. Applied Catalysis B: Environmental. 2008; 82(3-4): 180-189. doi: 10.1016/j.apcatb.2008.01.017 DOI: https://doi.org/10.1016/j.apcatb.2008.01.017
Sarker DR, Uddin MN, Elias M, et al. P-doped TiO2-MWCNTs nanocomposite thin films with enhanced photocatalytic activity under visible light exposure. Cleaner Engineering and Technology. 2022; 6: 100364. doi: 10.1016/j.clet.2021.100364 DOI: https://doi.org/10.1016/j.clet.2021.100364
Rao P, Wu D, Qin YY, et al. Facile fabrication of single-atom catalysts by a plasma-etching strategy for oxygen reduction reaction. Journal of Materials Chemistry A. 2022; 10(12): 6531-6537. doi: 10.1039/d1ta09154a DOI: https://doi.org/10.1039/D1TA09154A
Soares LG, Oliveira Vaz M de, Teixeira SR, et al. Absorbance determination and photocatalytic production of hydrogen using tungsten and TiO2 oxide nanostructures As catalyst. Cleaner Engineering and Technology. 2021; 5: 100268. doi: 10.1016/j.clet.2021.100268 DOI: https://doi.org/10.1016/j.clet.2021.100268
Rao P, Wu D, Luo J, et al. A plasma bombing strategy to synthesize high-loading single-atom catalysts for oxygen reduction reaction. Cell Reports Physical Science. 2022; 3(5): 100880. doi: 10.1016/j.xcrp.2022.100880 DOI: https://doi.org/10.1016/j.xcrp.2022.100880
Sitab AA, Tujjohra F, Rashid TU, et al. Thermally crosslinked electrospun nanofibrous mat from chrome-tanned solid wastes for cationic dye adsorption in wastewater treatment. Cleaner Engineering and Technology. 2023; 13: 100621. doi: 10.1016/j.clet.2023.100621 DOI: https://doi.org/10.1016/j.clet.2023.100621
Yao S, Ma Y, Xu T, et al. Ti–C bonds reinforced TiO2@C nanocomposite Na-ion battery electrodes by fluidized-bed plasma-enhanced chemical vapor deposition. Carbon. 2021; 171: 524-531. doi: 10.1016/j.carbon.2020.09.023 DOI: https://doi.org/10.1016/j.carbon.2020.09.023
Ho YM, Zheng WT, Li YA, et al. Field Emission Properties of Hybrid Carbon Nanotube−ZnO Nanoparticles. The Journal of Physical Chemistry C. 2008; 112(45): 17702-17708. doi: 10.1021/jp804566k DOI: https://doi.org/10.1021/jp804566k
Demidyuk V, Whitehead JC. Influence of Temperature on Gas-Phase Toluene Decomposition in Plasma-Catalytic System. Plasma Chemistry and Plasma Processing. 2006; 27(1): 85-94. doi: 10.1007/s11090-006-9045-z DOI: https://doi.org/10.1007/s11090-006-9045-z
Rao P, Wu D, Wang TJ, et al. Single atomic cobalt electrocatalyst for efficient oxygen reduction reaction. eScience. 2022; 2(4): 399-404. doi: 10.1016/j.esci.2022.05.004 DOI: https://doi.org/10.1016/j.esci.2022.05.004
Liang WJ, Ma L, Liu H, et al. Toluene degradation by non-thermal plasma combined with a ferroelectric catalyst. Chemosphere. 2013; 92(10): 1390-1395. doi: 10.1016/j.chemosphere.2013.05.042 DOI: https://doi.org/10.1016/j.chemosphere.2013.05.042
Luo Z, Lim S, You Y, et al. Effect of ion bombardment on the synthesis of vertically aligned single-walled carbon nanotubes by plasma-enhanced chemical vapor deposition. Nanotechnology. 2008; 19(25): 255607. doi: 10.1088/0957-4484/19/25/255607 DOI: https://doi.org/10.1088/0957-4484/19/25/255607
Yamazaki Y, Sakuma N, Katagiri M, et al. High-Quality Carbon Nanotube Growth at Low Temperature by Pulse-Excited Remote Plasma Chemical Vapor Deposition. Applied Physics Express. 2008; 1: 034004. doi: 10.1143/apex.1.034004 DOI: https://doi.org/10.1143/APEX.1.034004
Wang BB, Zheng K, Shao RW, et al. Structure and electrical property of gallium nitride nanowires synthesized in plasma-enhanced hot filament chemical vapor deposition system. Journal of Physics and Chemistry of Solids. 2013; 74(6): 862-866. doi: 10.1016/j.jpcs.2013.01.034 DOI: https://doi.org/10.1016/j.jpcs.2013.01.034
Sahoo G, Polaki SR, Ghosh S, et al. Plasma-tuneable oxygen functionalization of vertical graphenes enhance electrochemical capacitor performance. Energy Storage Materials. 2018; 14: 297-305. doi: 10.1016/j.ensm.2018.05.011 DOI: https://doi.org/10.1016/j.ensm.2018.05.011
Hussain S, Amade R, Jover E, et al. Growth and Plasma Functionalization of Carbon Nanotubes. Journal of Cluster Science. 2015; 26(2): 315-336. doi: 10.1007/s10876-015-0862-1 DOI: https://doi.org/10.1007/s10876-015-0862-1
Mehta P, Barboun P, Go DB, et al. Catalysis Enabled by Plasma Activation of Strong Chemical Bonds: A Review. ACS Energy Letters. 2019; 4(5): 1115-1133. doi: 10.1021/acsenergylett.9b00263 DOI: https://doi.org/10.1021/acsenergylett.9b00263
Wang W, Snoeckx R, Zhang X, et al. Modeling Plasma-based CO2 and CH4 Conversion in Mixtures with N2, O2, and H2O: The Bigger Plasma Chemistry Picture. The Journal of Physical Chemistry C. 2018; 122(16): 8704-8723. doi: 10.1021/acs.jpcc.7b10619 DOI: https://doi.org/10.1021/acs.jpcc.7b10619
Wang Q, Cheng Y, Jin Y. Dry reforming of methane in an atmospheric pressure plasma fluidized bed with Ni/γ-Al2O3 catalyst. Catalysis Today. 2009; 148(3-4): 275-282. doi: 10.1016/j.cattod.2009.08.008 DOI: https://doi.org/10.1016/j.cattod.2009.08.008
Wang Q, Yan BH, Jin Y, et al. Dry Reforming of Methane in a Dielectric Barrier Discharge Reactor with Ni/Al2O3Catalyst: Interaction of Catalyst and Plasma. Energy & Fuels. 2009; 23(8): 4196-4201. doi: 10.1021/ef900286j DOI: https://doi.org/10.1021/ef900286j
Sentek J, Krawczyk K, Młotek M, et al. Plasma-catalytic methane conversion with carbon dioxide in dielectric barrier discharges. Applied Catalysis B: Environmental. 2010; 94(1-2): 19-26. doi: 10.1016/j.apcatb.2009.10.016 DOI: https://doi.org/10.1016/j.apcatb.2009.10.016
Rico VJ, Hueso JL, Cotrino J, et al. Hybrid catalytic-DBD plasma reactor for the production of hydrogen and preferential CO oxidation (CO-PROX) at reduced temperatures. Chemical Communications. 2009; (41): 6192. doi: 10.1039/b909488a DOI: https://doi.org/10.1039/b909488a
Nozaki T, Muto N, Kado S, et al. Dissociation of vibrationally excited methane on Ni catalyst. Catalysis Today. 2004; 89(1-2): 57-65. doi: 10.1016/j.cattod.2003.11.040 DOI: https://doi.org/10.1016/j.cattod.2003.11.040
Di L, Xu Z, Wang K, et al. A facile method for preparing Pt/TiO2 photocatalyst with enhanced activity using dielectric barrier discharge. Catalysis Today. 2013; 211: 109-113. doi: 10.1016/j.cattod.2013.03.025 DOI: https://doi.org/10.1016/j.cattod.2013.03.025
Xu W, Zhang X, Dong M, et al. Plasma-assisted Ru/Zr-MOF catalyst for hydrogenation of CO2 to methane. Plasma Science and Technology. 2019; 21(4): 044004. doi: 10.1088/2058-6272/aaf9d2 DOI: https://doi.org/10.1088/2058-6272/aaf9d2
Li Y, Wei Z, Wang Y. Ni/MgO catalyst prepared via dielectric-barrier discharge plasma with improved catalytic performance for carbon dioxide reforming of methane. Frontiers of Chemical Science and Engineering. 2014; 8(2): 133-140. doi: 10.1007/s11705-014-1422-1 DOI: https://doi.org/10.1007/s11705-014-1422-1
Gallon HJ, Tu X, Twigg MV, et al. Plasma-assisted methane reduction of a NiO catalyst—Low temperature activation of methane and formation of carbon nanofibres. Applied Catalysis B: Environmental. 2011; 106(3-4): 616-620. doi: 10.1016/j.apcatb.2011.06.023 DOI: https://doi.org/10.1016/j.apcatb.2011.06.023
Tu X, Gallon HJ, Whitehead JC. Plasma-assisted reduction of a NiO/Al2O3 catalyst in atmospheric pressure H2/Ar dielectric barrier discharge. Catalysis Today. 2013; 211: 120-125. doi: 10.1016/j.cattod.2013.03.024 DOI: https://doi.org/10.1016/j.cattod.2013.03.024
Tu X, Gallon HJ, Whitehead JC. Electrical and spectroscopic diagnostics of a single-stage plasma-catalysis system: effect of packing with TiO2. Journal of Physics D: Applied Physics. 2011; 44(48): 482003. doi: 10.1088/0022-3727/44/48/482003 DOI: https://doi.org/10.1088/0022-3727/44/48/482003
Xu W, Zhan Z, Di L, et al. Enhanced activity for CO oxidation over Pd/Al2O3 catalysts prepared by atmospheric-pressure cold plasma. Catalysis Today. 2015; 256: 148-152. doi: 10.1016/j.cattod.2015.01.017 DOI: https://doi.org/10.1016/j.cattod.2015.01.017
Di L, Zhan Z, Zhang X, et al. Atmospheric-Pressure DBD Cold Plasma for Preparation of High Active Au/P25 Catalysts for Low-Temperature CO Oxidation. Plasma Science and Technology. 2016; 18(5): 544-548. doi: 10.1088/1009-0630/18/5/17 DOI: https://doi.org/10.1088/1009-0630/18/5/17
Mizushima T, Matsumoto K, Sugoh J, et al. Tubular membrane-like catalyst for reactor with dielectric-barrier-discharge plasma and its performance in ammonia synthesis. Applied Catalysis A: General. 2004; 265(1): 53-59. doi: 10.1016/j.apcata.2004.01.002 DOI: https://doi.org/10.1016/j.apcata.2004.01.002
Mizushima T, Matsumoto K, Ohkita H, et al. Catalytic Effects of Metal-loaded Membrane-like Alumina Tubes on Ammonia Synthesis in Atmospheric Pressure Plasma by Dielectric Barrier Discharge. Plasma Chemistry and Plasma Processing. 2006; 27(1): 1-11. doi: 10.1007/s11090-006-9034-2 DOI: https://doi.org/10.1007/s11090-006-9034-2
Yu S, Liang Y, Sun S, et al. Vehicle Exhaust Gas Clearance by Low Temperature Plasma-Driven Nano-Titanium Dioxide Film Prepared by Radiofrequency Magnetron Sputtering. Marr AC, ed. PLoS ONE. 2013; 8(4): e59974. doi: 10.1371/journal.pone.0059974 DOI: https://doi.org/10.1371/journal.pone.0059974
Hou WC, Chen LY, Hong FCN. Fabrication of gallium nitride nanowires by nitrogen plasma. Diamond and Related Materials. 2008; 17(7-10): 1780-1784. doi: 10.1016/j.diamond.2008.02.003 DOI: https://doi.org/10.1016/j.diamond.2008.02.003
Mei D, Zhu X, He YL, et al. Plasma-assisted conversion of CO2 in a dielectric barrier discharge reactor: understanding the effect of packing materials. Plasma Sources Science and Technology. 2014; 24(1): 015011. doi: 10.1088/0963-0252/24/1/015011 DOI: https://doi.org/10.1088/0963-0252/24/1/015011
Yu Q, Kong M, Liu T, et al. Characteristics of the Decomposition of CO2 in a Dielectric Packed-Bed Plasma Reactor. Plasma Chemistry and Plasma Processing. 2011; 32(1): 153-163. doi: 10.1007/s11090-011-9335-y DOI: https://doi.org/10.1007/s11090-011-9335-y
Scapinello M, Martini LM, Tosi P. CO2 Hydrogenation by CH4in a Dielectric Barrier Discharge: Catalytic Effects of Nickel and Copper. Plasma Processes and Polymers. 2014; 11(7): 624-628. doi: 10.1002/ppap.201400023 DOI: https://doi.org/10.1002/ppap.201400023
Krawczyk K, Młotek M, Ulejczyk B, et al. Methane conversion with carbon dioxide in plasma-catalytic system. Fuel. 2014; 117: 608-617. doi: 10.1016/j.fuel.2013.08.068 DOI: https://doi.org/10.1016/j.fuel.2013.08.068
Spencer LF, Gallimore AD. CO2 dissociation in an atmospheric pressure plasma/catalyst system: a study of efficiency. Plasma Sources Science and Technology. 2012; 22(1): 015019. doi: 10.1088/0963-0252/22/1/015019 DOI: https://doi.org/10.1088/0963-0252/22/1/015019
Pietruszka B, Heintze M. Methane conversion at low temperature: the combined application of catalysis and non-equilibrium plasma. Catalysis Today. 2004; 90(1-2): 151-158. doi: 10.1016/j.cattod.2004.04.021 DOI: https://doi.org/10.1016/j.cattod.2004.04.021
Tu X, Gallon HJ, Twigg MV, et al. Dry reforming of methane over a Ni/Al2O3 catalyst in a coaxial dielectric barrier discharge reactor. Journal of Physics D: Applied Physics. 2011; 44(27): 274007. doi: 10.1088/0022-3727/44/27/274007 DOI: https://doi.org/10.1088/0022-3727/44/27/274007
Chen L, Zhang XW, Huang L, et al. Partial oxidation of methane with air for methanol production in a post-plasma catalytic system. Chemical Engineering and Processing: Process Intensification. 2009; 48(8): 1333-1340. doi: 10.1016/j.cep.2009.06.007 DOI: https://doi.org/10.1016/j.cep.2009.06.007
Li Z. Plasma treatment of Ni catalyst via a corona discharge. Journal of Molecular Catalysis A: Chemical. 2004; 211(1-2): 149-153. doi: 10.1016/j.molcata.2003.10.003
Dong P, Yang F, Cheng X, et al. Plasmon enhanced photocatalytic and antimicrobial activities of Ag-TiO2 nanocomposites under visible light irradiation prepared by DBD cold plasma treatment. Materials Science and Engineering: C. 2019; 96: 197-204. doi: 10.1016/j.msec.2018.11.005 DOI: https://doi.org/10.1016/j.msec.2018.11.005
Tu X, Whitehead JC. Plasma-catalytic dry reforming of methane in an atmospheric dielectric barrier discharge: Understanding the synergistic effect at low temperature. Applied Catalysis B: Environmental. 2012; 125: 439-448. doi: 10.1016/j.apcatb.2012.06.006 DOI: https://doi.org/10.1016/j.apcatb.2012.06.006
Chen P, Tao L, Xie Y. Non-thermal plasma cooperating catalyst degradation of the volatile organic compounds: A review. Chemical Industry and Engineering Progress. 2019; 38(9): 4284-4294. doi: 10.16085/j.issn.1000-6613.2018-2485
Shang S, Liu G, Chai X, et al. Research on Ni/γ-Al2O3 catalyst for CO2 reforming of CH4 prepared by atmospheric pressure glow discharge plasma jet. Catalysis Today. 2009; 148(3-4): 268-274. doi: 10.1016/j.cattod.2009.09.011 DOI: https://doi.org/10.1016/j.cattod.2009.09.011
Weltmann K ‐D., Kindel E, Brandenburg R, et al. Atmospheric Pressure Plasma Jet for Medical Therapy: Plasma Parameters and Risk Estimation. Contributions to Plasma Physics. 2009; 49(9): 631-640. doi: 10.1002/ctpp.200910067 DOI: https://doi.org/10.1002/ctpp.200910067
Reuter S, von Woedtke T, Weltmann KD. The kINPen—a review on physics and chemistry of the atmospheric pressure plasma jet and its applications. Journal of Physics D: Applied Physics. 2018; 51(23): 233001. doi: 10.1088/1361-6463/aab3ad
Sajjadi SM, Haghighi M. Influence of tungsten loading on CO2/O2 reforming of methane over Co-W-promoted NiO-Al2 O3 nanocatalyst designed by sol-gel-plasma. International Journal of Energy Research. 2018; 43(2): 853-873. doi: 10.1002/er.4316 DOI: https://doi.org/10.1002/er.4316
Shimizu K, Kaneta S, Blajan M, et al. Surface modification of dye-sensitized solid-state solar cells by atmospheric-pressure plasma jet. Japanese Journal of Applied Physics. 2014; 53(11S): 11RF02. doi: 10.7567/jjap.53.11rf02 DOI: https://doi.org/10.7567/JJAP.53.11RF02
Chang S, Rodríguez Tolava EF, Yang Y, et al. One‐Step Fast Synthesis of Li4Ti5O12 Particles Using an Atmospheric Pressure Plasma Jet. Dunn B, ed. Journal of the American Ceramic Society. 2013; 97(3): 708-712. doi: 10.1111/jace.12728 DOI: https://doi.org/10.1111/jace.12728
Kang M, Kim B J, Cho S M, 2002. Decomposition of Toluene Using an Atmospheric Pressure Plasma/TiO2 Catalytic System. Journal of Molecular Catalysis A: Chemical. 180: 125-132. doi: 10.1016/S1381-1169(01)00417-4 DOI: https://doi.org/10.1016/S1381-1169(01)00417-4
Rahemi N, Haghighi M, Babaluo AA, et al. Synthesis and physicochemical characterizations of Ni/Al2O3–ZrO2 nanocatalyst prepared via impregnation method and treated with non-thermal plasma for CO2 reforming of CH4. Journal of Industrial and Engineering Chemistry. 2013; 19(5): 1566-1576. doi: 10.1016/j.jiec.2013.01.024 DOI: https://doi.org/10.1016/j.jiec.2013.01.024
Hofmann S, Ducati C, Neill RJ, et al. Gold catalyzed growth of silicon nanowires by plasma enhanced chemical vapor deposition. Journal of Applied Physics. 2003; 94(9): 6005-6012. doi: 10.1063/1.1614432 DOI: https://doi.org/10.1063/1.1614432
Li Z. Plasma treatment of Ni catalyst via a corona discharge. Journal of Molecular Catalysis A: Chemical. 2004; 211(1-2): 149-153. doi: 10.1016/j.molcata.2003.10.003 DOI: https://doi.org/10.1016/j.molcata.2003.10.003
Jiang N, Shang KF, Lu N, et al. High-Efficiency Removal of NOx From Flue Gas by Multitooth Wheel-Cylinder Corona Discharge Plasma Facilitated Selective Catalytic Reduction Process. IEEE Transactions on Plasma Science. 2016; 44(11): 2738-2744. doi: 10.1109/tps.2016.2609140 DOI: https://doi.org/10.1109/TPS.2016.2609140
Lu N, Wang C, Lou C. Remediation of PAH-contaminated soil by pulsed corona discharge plasma. Journal of Soils and Sediments. 2016; 17(1): 97-105. doi: 10.1007/s11368-016-1473-7 DOI: https://doi.org/10.1007/s11368-016-1473-7
Zhang M, Sun Y, Jiang H, et al. Degradation of dimethyl phthalate in aqueous by corona discharge plasma combined with a Bi2WO6 nano-catalyst. Acta Scientiae Circumstantiae. 2016; 36(5): 1690-1696.
Zhao B, Liu Y, Zhu Z, et al. Highly selective conversion of CO2 into ethanol on Cu/ZnO/Al2O3 catalyst with the assistance of plasma. Journal of CO2 Utilization. 2018; 24: 34-39. doi: 10.1016/j.jcou.2017.10.013 DOI: https://doi.org/10.1016/j.jcou.2017.10.013
Zhang X, Zhu A, Li X, et al. Oxidative dehydrogenation of ethane with CO2 over catalyst under pulse corona plasma. Catalysis Today. 2004; 89(1-2): 97-102. doi: 10.1016/j.cattod.2003.11.015 DOI: https://doi.org/10.1016/j.cattod.2003.11.015
Saud S, Nguyen DB, Kim SG, et al. Improvement of Ethylene Removal Performance by Adsorption/Oxidation in a Pin-Type Corona Discharge Coupled with Pd/ZSM-5 Catalyst. Catalysts. 2020; 10(1): 133. doi: 10.3390/catal10010133 DOI: https://doi.org/10.3390/catal10010133
Chao Y. Hydrogen production via partial oxidation of methane with plasma-assisted catalysis. International Journal of Hydrogen Energy. 2008; 33(2): 664-671. doi: 10.1016/j.ijhydene.2007.09.024 DOI: https://doi.org/10.1016/j.ijhydene.2007.09.024
Yu X, Shen X, Li J, et al. Catalytic degradation of butyl acetate by low-temperature plasma coupling (Chinese). Environmental Protection and Circular Economy. 2020; 8(4):11-14. doi: 10.3969/j.issn.1674-1021.2020.08.004
Aziznia A, Bozorgzadeh H R, Seyed-Matin N, 2012. Comparison of Dry Reforming of Methane in Low Temperature Hybrid Plasma-Catalytic Corona with Thermal Catalytic Reactor over Ni/gamma-Al2O3. Journal of Natural Gas Chemistry. 21(4): 466-475. doi: 10.1016/S1003-9953(11)60392-7 DOI: https://doi.org/10.1016/S1003-9953(11)60392-7
Chavadej S, Kiatubolpaiboon W, Rangsunvigit P, et al. A combined multistage corona discharge and catalytic system for gaseous benzene removal. Journal of Molecular Catalysis A: Chemical. 2007; 263(1-2): 128-136. doi: 10.1016/j.molcata.2006.08.061 DOI: https://doi.org/10.1016/j.molcata.2006.08.061
Marafee A, Liu C, Xu G, et al. An Experimental Study on the Oxidative Coupling of Methane in a Direct Current Corona Discharge Reactor over Sr/La2O3 Catalyst. Industrial & Engineering Chemistry Research. 1997; 36(3): 632-637. doi: 10.1021/ie960139b DOI: https://doi.org/10.1021/ie960139b
Reuter S, von Woedtke T, Weltmann KD. The kINPen—a review on physics and chemistry of the atmospheric pressure plasma jet and its applications. Journal of Physics D: Applied Physics. 2018; 51(23): 233001. doi: 10.1088/1361-6463/aab3ad DOI: https://doi.org/10.1088/1361-6463/aab3ad
Sergiienko R, Shibata E, Zentaro A, et al. Formation and characterization of graphite-encapsulated cobalt nanoparticles synthesized by electric discharge in an ultrasonic cavitation field of liquid ethanol. Acta Materialia. 2007; 55(11): 3671-3680. doi: 10.1016/j.actamat.2007.02.017 DOI: https://doi.org/10.1016/j.actamat.2007.02.017
Show Y, Hirai A, Almowarai A, et al. Platinum catalyst formed on carbon nanotube by the in-liquid plasma method for fuel cell. Thin Solid Films. 2015; 596: 198-200. doi: 10.1016/j.tsf.2015.08.053 DOI: https://doi.org/10.1016/j.tsf.2015.08.053
Bulychev NA, Kazaryan MA, Chaikov LL, et al. Nanoscale metal oxide particles produced in the plasma discharge in the liquid phase upon exposure to ultrasonic cavitation. 1. Method for producing particles. Bulletin of the Lebedev Physics Institute. 2014; 41(9): 264-268. doi: 10.3103/s106833561409005x DOI: https://doi.org/10.3103/S106833561409005X
Hattori Y, Nomura S, Mukasa S, et al. Synthesis of tungsten oxide, silver, and gold nanoparticles by radio frequency plasma in water. Journal of Alloys and Compounds. 2013; 578: 148-152. doi: 10.1016/j.jallcom.2013.05.032 DOI: https://doi.org/10.1016/j.jallcom.2013.05.032
Xue H, Yang T, Zhang Z, et al. Stimulate the hidden catalysis potential and exposure of nickel site in NiSe@CNTs result in ultra-high HER/OER activity and stability. Applied Catalysis B: Environmental. 2023; 330: 122641. doi: 10.1016/j.apcatb.2023.122641 DOI: https://doi.org/10.1016/j.apcatb.2023.122641
Wang J, Yang T, Li X, et al. Hydrogen evolution reaction activity enhancement from active site turnover mechanism. Journal of Energy Chemistry. 2024; 92: 629-638. doi: 10.1016/j.jechem.2024.02.006 DOI: https://doi.org/10.1016/j.jechem.2024.02.006
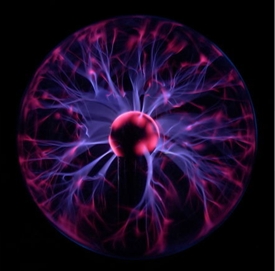
Downloads
Published
How to Cite
Issue
Section
License
Copyright (c) 2024 Qingbin Tian, Lansen Bi, Shuyan Lin, Jiangshan Gao, Yan He

This work is licensed under a Creative Commons Attribution 4.0 International License.