A brief review of hydrogen production technologies
DOI:
https://doi.org/10.18686/cest.v2i1.117Keywords:
hydrogen energy; new energy utilization; hydrogen production technology; renewable energyAbstract
As a result of the array of problems arising from the use of fossil fuels, it is necessary to develop and optimize alternative energy technologies. Despite hydrogen being an ideal form of energy, its primary source is still fossil fuels via conventional methods. Therefore, several hydrogen-production resources and techniques have been investigated, providing feasibility for clean and effective hydrogen production. This paper provided a mini-review of hydrogen production technologies, including renewable energy, chemical looping, water electrolysis, photocatalysis, and plasma.
References
1. Cui P, Yao D, Ma Z, et al. Life cycle water footprint comparison of biomass-to-hydrogen and coal-to-hydrogen processes. Science of The Total Environment. 2021; 773: 145056. doi: 10.1016/j.scitotenv.2021.145056 DOI: https://doi.org/10.1016/j.scitotenv.2021.145056
2. Liu W, Liu C, Gogoi P, et al. Overview of Biomass Conversion to Electricity and Hydrogen and Recent Developments in Low-Temperature Electrochemical Approaches. Engineering. 2020; 6(12): 1351-1363. doi: 10.1016/j.eng.2020.02.021 DOI: https://doi.org/10.1016/j.eng.2020.02.021
3. Ahmadi MH, Jashnani H, Chau KW, et al. Carbon dioxide emissions prediction of five Middle Eastern countries using artificial neural networks. Energy Sources, Part A: Recovery, Utilization, and Environmental Effects. 2019; 45(3): 9513-9525. doi: 10.1080/15567036.2019.1679914 DOI: https://doi.org/10.1080/15567036.2019.1679914
4. Davis SJ, Lewis NS, Shaner M, et al. Net-zero emissions energy systems. Science. 2018; 360(6396). doi: 10.1126/science.aas9793 DOI: https://doi.org/10.1126/science.aas9793
5. Boyd PG, Chidambaram A, García-Díez E, et al. Data-driven design of metal–organic frameworks for wet flue gas CO2 capture. Nature. 2019; 576(7786): 253-256. doi: 10.1038/s41586-019-1798-7 DOI: https://doi.org/10.1038/s41586-019-1798-7
6. Kumar R, Ahmadi MH, Rajak DK, et al. A study on CO2 absorption using hybrid solvents in packed columns. International Journal of Low-Carbon Technologies. Published online October 14, 2019. doi: 10.1093/ijlct/ctz051 DOI: https://doi.org/10.1093/ijlct/ctz051
7. Roh HS. Nanocatalysts for Hydrogen Production. Catalysts. 2021; 11(2): 288. doi: 10.3390/catal11020288 DOI: https://doi.org/10.3390/catal11020288
8. Xu X, Zhou Q, Yu D. The future of hydrogen energy: Bio-hydrogen production technology. International Journal of Hydrogen Energy. 2022; 47(79): 33677-33698. doi: 10.1016/j.ijhydene.2022.07.261 DOI: https://doi.org/10.1016/j.ijhydene.2022.07.261
9. Dawood F, Anda M, Shafiullah GM. Hydrogen production for energy: An overview. International Journal of Hydrogen Energy. 2020; 45(7): 3847-3869. doi: 10.1016/j.ijhydene.2019.12.059 DOI: https://doi.org/10.1016/j.ijhydene.2019.12.059
10. Midilli A, Ay M, Dincer I, et al. On hydrogen and hydrogen energy strategies. Renewable and Sustainable Energy Reviews. 2005; 9(3): 255-271. doi: 10.1016/j.rser.2004.05.003 DOI: https://doi.org/10.1016/j.rser.2004.05.003
11. Tadlock T, Rubio O, Ristanovic D. The Green Hydrogen Revolution - Integrating Hydrogen Into Industrial Applications. 2022 IEEE IAS Petroleum and Chemical Industry Technical Conference (PCIC). Published online September 26, 2022. doi: 10.1109/pcic42668.2022.10181257 DOI: https://doi.org/10.1109/PCIC42668.2022.10181257
12. e Silva MPG da C, Miranda JC de C. Exergy efficiency of thermochemical syngas-to-ethanol production plants. SN Applied Sciences. 2021; 3(5). doi: 10.1007/s42452-021-04526-3 DOI: https://doi.org/10.1007/s42452-021-04526-3
13. Gao Y, Jausseme C, Huang Z, et al. Hydrogen-Powered Aircraft: Hydrogen–electric hybrid propulsion for aviation. IEEE Electrification Magazine. 2022; 10(2): 17-26. doi: 10.1109/mele.2022.3165725 DOI: https://doi.org/10.1109/MELE.2022.3165725
14. Huang J, Balcombe P, Feng Z. Technical and economic analysis of different colours of producing hydrogen in China. Fuel. 2023; 337: 127227. doi: 10.1016/j.fuel.2022.127227 DOI: https://doi.org/10.1016/j.fuel.2022.127227
15. Jang D, Kim K, Kim KH, et al. Techno-economic analysis and Monte Carlo simulation for green hydrogen production using offshore wind power plant. Energy Conversion and Management. 2022; 263: 115695. doi: 10.1016/j.enconman.2022.115695 DOI: https://doi.org/10.1016/j.enconman.2022.115695
16. Karayel GK, Javani N, Dincer I. Hydropower for green hydrogen production in Turkey. International Journal of Hydrogen Energy. 2023; 48(60): 22806-22817. doi: 10.1016/j.ijhydene.2022.04.084 DOI: https://doi.org/10.1016/j.ijhydene.2022.04.084
17. Shiva Kumar S, Lim H. An overview of water electrolysis technologies for green hydrogen production. Energy Reports. 2022; 8: 13793-13813. doi: 10.1016/j.egyr.2022.10.127 DOI: https://doi.org/10.1016/j.egyr.2022.10.127
18. Mazzeo D, Herdem MS, Matera N, et al. Green hydrogen production: Analysis for different single or combined large-scale photovoltaic and wind renewable systems. Renewable Energy. 2022; 200: 360-378. doi: 10.1016/j.renene.2022.09.057 DOI: https://doi.org/10.1016/j.renene.2022.09.057
19. Li Z, Zhang W, Zhang R, et al. Development of renewable energy multi-energy complementary hydrogen energy system (A Case Study in China): A review. Energy Exploration & Exploitation. 2020; 38(6): 2099-2127. doi: 10.1177/0144598720953512 DOI: https://doi.org/10.1177/0144598720953512
20. Song HG, Chun YN. Tar decomposition-reforming conversion on microwave-heating carbon receptor. Energy. 2020; 199: 117482. doi: 10.1016/j.energy.2020.117482 DOI: https://doi.org/10.1016/j.energy.2020.117482
21. Li Q, Wang Q, Kayamori A, et al. Experimental study and modeling of heavy tar steam reforming. Fuel Processing Technology. 2018; 178: 180-188. doi: 10.1016/j.fuproc.2018.05.020 DOI: https://doi.org/10.1016/j.fuproc.2018.05.020
22. Tan RS, Tuan Abdullah TA, Johari A, et al. Catalytic steam reforming of tar for enhancing hydrogen production from biomass gasification: a review. Frontiers in Energy. 2020; 14(3): 545-569. doi: 10.1007/s11708-020-0800-2 DOI: https://doi.org/10.1007/s11708-020-0800-2
23. Yang H, Yan R, Chen H, et al. Characteristics of hemicellulose, cellulose and lignin pyrolysis. Fuel. 2007; 86(12-13): 1781-1788. doi: 10.1016/j.fuel.2006.12.013 DOI: https://doi.org/10.1016/j.fuel.2006.12.013
24. Quan C, Gao N, Song Q. Pyrolysis of biomass components in a TGA and a fixed-bed reactor: Thermochemical behaviors, kinetics, and product characterization. Journal of Analytical and Applied Pyrolysis. 2016; 121: 84-92. doi: 10.1016/j.jaap.2016.07.005 DOI: https://doi.org/10.1016/j.jaap.2016.07.005
25. Yang J, Rizkiana J, Widayatno WB, et al. Fast co-pyrolysis of low density polyethylene and biomass residue for oil production. Energy Conversion and Management. 2016; 120: 422-429. doi: 10.1016/j.enconman.2016.05.008 DOI: https://doi.org/10.1016/j.enconman.2016.05.008
26. Chen YH, Schmid M, Kertthong T, et al. Reforming of toluene as a tar model compound over straw char containing fly ash. Biomass and Bioenergy. 2020; 141: 105657. doi: 10.1016/j.biombioe.2020.105657 DOI: https://doi.org/10.1016/j.biombioe.2020.105657
27. Trinh QT, Nguyen AV, Huynh DC, et al. Mechanistic insights into the catalytic elimination of tar and the promotional effect of boron on it: first-principles study using toluene as a model compound. Catalysis Science & Technology. 2016; 6(15): 5871-5883. doi: 10.1039/c6cy00358c DOI: https://doi.org/10.1039/C6CY00358C
28. Mukai D, Murai Y, Higo T, et al. In situ IR study for elucidating reaction mechanism of toluene steam reforming over Ni/La0.7Sr0.3AlO3−δ catalyst. Applied Catalysis A: General. 2013; 466: 190-197. doi: 10.1016/j.apcata.2013.06.052 DOI: https://doi.org/10.1016/j.apcata.2013.06.052
29. Xie W, Yang J, Wang Q, et al. Layered perovskite-like La2−xCaxNiO4±δ derived catalysts for hydrogen production via auto-thermal reforming of acetic acid. Catalysis Science & Technology. 2018; 8(12): 3015-3024. doi: 10.1039/c8cy00116b DOI: https://doi.org/10.1039/C8CY00116B
30. Goicoechea S, Ehrich H, Arias PL, et al. Thermodynamic analysis of acetic acid steam reforming for hydrogen production. Journal of Power Sources. 2015; 279: 312-322. doi: 10.1016/j.jpowsour.2015.01.012 DOI: https://doi.org/10.1016/j.jpowsour.2015.01.012
31. Hoang TMC, Geerdink B, Sturm JM, et al. Steam reforming of acetic acid – A major component in the volatiles formed during gasification of humin. Applied Catalysis B: Environmental. 2015; 163: 74-82. doi: 10.1016/j.apcatb.2014.07.046 DOI: https://doi.org/10.1016/j.apcatb.2014.07.046
32. Wang S, Li X, Zhang F, et al. Bio-oil catalytic reforming without steam addition: Application to hydrogen production and studies on its mechanism. International Journal of Hydrogen Energy. 2013; 38(36): 16038-16047. doi: 10.1016/j.ijhydene.2013.10.032 DOI: https://doi.org/10.1016/j.ijhydene.2013.10.032
33. Lewis WK, Gilliland ER, Reed WA. Reaction of Methane with Copper Oxide in a Fluidized Bed. Industrial & Engineering Chemistry. 1949; 41(6): 1227-1237. doi: 10.1021/ie50474a018 DOI: https://doi.org/10.1021/ie50474a018
34. Güleç F, Okolie JA. Decarbonising bioenergy through biomass utilisation in chemical looping combustion and gasification: a review. Environmental Chemistry Letters. 2023; 22(1): 121-147. doi: 10.1007/s10311-023-01656-5 DOI: https://doi.org/10.1007/s10311-023-01656-5
35. Das S, Biswas A, Tiwary CS, et al. Hydrogen production using chemical looping technology: A review with emphasis on H2 yield of various oxygen carriers. International Journal of Hydrogen Energy. 2022; 47(66): 28322-28352. doi: 10.1016/j.ijhydene.2022.06.170 DOI: https://doi.org/10.1016/j.ijhydene.2022.06.170
36. Kang KS, Kim CH, Bae KK, et al. Oxygen-carrier selection and thermal analysis of the chemical-looping process for hydrogen production. International Journal of Hydrogen Energy. 2010; 35(22): 12246-12254. doi: 10.1016/j.ijhydene.2010.08.043 DOI: https://doi.org/10.1016/j.ijhydene.2010.08.043
37. Kobayashi N, Itaya Y. Performance of iron oxide-based oxygen carrier in biomass pyrolysis. Journal of Chemical Engineering of Japan. 2018; 51(5): 469-475. doi: 10.1252/jcej.17we111 DOI: https://doi.org/10.1252/jcej.17we111
38. Chang Y, Li G, Ma S, et al. Effect of hierarchical pore structure of oxygen carrier on the performance of biomass chemical looping hydrogen generation. Energy. 2022; 254: 124301. doi: 10.1016/j.energy.2022.124301 DOI: https://doi.org/10.1016/j.energy.2022.124301
39. Zeng DW, Peng S, Chen C, et al. Nanostructured Fe2O3/MgAl2O4 material prepared by colloidal crystal templated sol–gel method for chemical looping with hydrogen storage. International Journal of Hydrogen Energy. 2016; 41(48): 22711-22721. doi: 10.1016/j.ijhydene.2016.09.180 DOI: https://doi.org/10.1016/j.ijhydene.2016.09.180
40. Liu YC, Ku Y, Tseng YH, et al. Fabrication of Fe2O3/TiO2 Oxygen Carriers for Chemical Looping Combustion and Hydrogen Generation. Aerosol and Air Quality Research. 2016; 16(8): 2023-2032. doi: 10.4209/aaqr.2015.10.0603 DOI: https://doi.org/10.4209/aaqr.2015.10.0603
41. Shimokawa K, Atsumi T, Harada M, et al. Zinc-based spinel cathode materials for magnesium rechargeable batteries: toward the reversible spinel–rocksalt transition. Journal of Materials Chemistry A. 2019; 7(19): 12225-12235. doi: 10.1039/c9ta02281c DOI: https://doi.org/10.1039/C9TA02281C
42. Hu Q, Shen Y, Chew JW, et al. Chemical looping gasification of biomass with Fe2O3/CaO as the oxygen carrier for hydrogen-enriched syngas production. Chemical Engineering Journal. 2020; 379: 122346. doi: 10.1016/j.cej.2019.122346 DOI: https://doi.org/10.1016/j.cej.2019.122346
43. Liu C, Luo J, Dong H, et al. Hydrogen-rich syngas production form biomass char by chemical looping gasification with Fe/Ca-based oxygen carrier. Separation and Purification Technology. 2022; 300: 121912. doi: 10.1016/j.seppur.2022.121912 DOI: https://doi.org/10.1016/j.seppur.2022.121912
44. Huang Z, Deng Z, Chen D, He F, Li H. Performance of NiFe2O4 oxygen carrier in hydrogen production by chemical looping steam reforming. Modern Chemical Industry. 2018; 38(9): 90-95. doi: 10.16606/j.cnki.issn0253-4320.2018.09.021
45. Gao J, Pu G, Wang P, et al. Study on the reaction performance of Ce‐doped NiFe2O4 oxygen carriers in the process of chemical looping hydrogen production. International Journal of Energy Research. 2021; 46(3): 2810-2825. doi: 10.1002/er.7346 DOI: https://doi.org/10.1002/er.7346
46. Wang Y, Zhu Q, Xie T, et al. Promoted Alkaline Hydrogen Evolution Reaction Performance of Ru/C by Introducing TiO2 Nanoparticles. ChemElectroChem. 2020; 7(5): 1182-1186. doi: 10.1002/celc.201902170 DOI: https://doi.org/10.1002/celc.201902170
47. Guo JX, Yan DY, Qiu KW, et al. High electrocatalytic hydrogen evolution activity on a coupled Ru and CoO hybrid electrocatalyst. Journal of Energy Chemistry. 2019; 37: 143-147. doi: 10.1016/j.jechem.2018.12.011 DOI: https://doi.org/10.1016/j.jechem.2018.12.011
48. Li R, Kuang P, Wageh S, et al. Potential-dependent reconstruction of Ni-based cuboid arrays for highly efficient hydrogen evolution coupled with electro-oxidation of organic compound. Chemical Engineering Journal. 2023; 453: 139797. doi: 10.1016/j.cej.2022.139797 DOI: https://doi.org/10.1016/j.cej.2022.139797
49. Li J, Xu P, Zhou R, et al. Co9S8–Ni3S2 heterointerfaced nanotubes on Ni foam as highly efficient and flexible bifunctional electrodes for water splitting. Electrochimica Acta. 2019; 299: 152-162. doi: 10.1016/j.electacta.2019.01.001 DOI: https://doi.org/10.1016/j.electacta.2019.01.001
50. An L, Wei C, Lu M, et al. Recent Development of Oxygen Evolution Electrocatalysts in Acidic Environment. Advanced Materials. 2021; 33(20). doi: 10.1002/adma.202006328 DOI: https://doi.org/10.1002/adma.202006328
51. Fukazawa A, Tanaka K, Hashimoto Y, et al. Electrocatalytic asymmetric hydrogenation of α,β-unsaturated acids in a PEM reactor with cinchona-modified palladium catalysts. Electrochemistry Communications. 2020; 115: 106734. doi: 10.1016/j.elecom.2020.106734 DOI: https://doi.org/10.1016/j.elecom.2020.106734
52. Vincent I, Bessarabov D. Low cost hydrogen production by anion exchange membrane electrolysis: A review. Renewable and Sustainable Energy Reviews. 2018; 81: 1690-1704. doi: 10.1016/j.rser.2017.05.258 DOI: https://doi.org/10.1016/j.rser.2017.05.258
53. Li C, Baek JB. The promise of hydrogen production from alkaline anion exchange membrane electrolyzers. Nano Energy. 2021; 87: 106162. doi: 10.1016/j.nanoen.2021.106162 DOI: https://doi.org/10.1016/j.nanoen.2021.106162
54. Ham K, Hong S, Kang S, et al. Extensive Active-Site Formation in Trirutile CoSb2O6 by Oxygen Vacancy for Oxygen Evolution Reaction in Anion Exchange Membrane Water Splitting. ACS Energy Letters. 2021; 6(2): 364-370. doi: 10.1021/acsenergylett.0c02359 DOI: https://doi.org/10.1021/acsenergylett.0c02359
55. Lim A, Kim HJ, Henkensmeier D, et al. A study on electrode fabrication and operation variables affecting the performance of anion exchange membrane water electrolysis. Journal of Industrial and Engineering Chemistry. 2019; 76: 410-418. doi: 10.1016/j.jiec.2019.04.007 DOI: https://doi.org/10.1016/j.jiec.2019.04.007
56. Varcoe JR, Atanassov P, Dekel DR, et al. Anion-exchange membranes in electrochemical energy systems. Energy Environ Sci. 2014; 7(10): 3135-3191. doi: 10.1039/c4ee01303d DOI: https://doi.org/10.1039/C4EE01303D
57. Mandal M. Recent Advancement on Anion Exchange Membranes for Fuel Cell and Water Electrolysis. ChemElectroChem. 2020; 8(1): 36-45. doi: 10.1002/celc.202001329 DOI: https://doi.org/10.1002/celc.202001329
58. Jiang Z, Yi G, Yao X, et al. Durable and highly-efficient anion exchange membrane water electrolysis using poly(biphenyl alkylene) membrane. Chemical Engineering Journal. 2023; 467: 143442. doi: 10.1016/j.cej.2023.143442 DOI: https://doi.org/10.1016/j.cej.2023.143442
59. Nechache A, Hody S. Alternative and innovative solid oxide electrolysis cell materials: A short review. Renewable and Sustainable Energy Reviews. 2021; 149: 111322. doi: 10.1016/j.rser.2021.111322 DOI: https://doi.org/10.1016/j.rser.2021.111322
60. Zheng Y, Chen Z, Zhang J. Solid Oxide Electrolysis of H2O and CO2 to Produce Hydrogen and Low-Carbon Fuels. Electrochemical Energy Reviews. 2021; 4(3): 508-517. doi: 10.1007/s41918-021-00097-4 DOI: https://doi.org/10.1007/s41918-021-00097-4
61. Liu RT, Xu ZL, Li FM, et al. Recent advances in proton exchange membrane water electrolysis. Chemical Society Reviews. 2023; 52(16): 5652-5683. doi: 10.1039/d2cs00681b DOI: https://doi.org/10.1039/D2CS00681B
62. Cao J, Zhang W, Li Y, et al. Current status of hydrogen production in China. Progress in Chemistry. 2021; 33(12): 2215-2244. doi: 10.7536/PC201128
63. Tonks L, Langmuir I. A General Theory of the Plasma of an Arc. Physical Review. 1929; 34(6): 876-922. doi: 10.1103/physrev.34.876 DOI: https://doi.org/10.1103/PhysRev.34.876
64. Liu L, Zhang Z, Das S, et al. LaNiO3 as a precursor of Ni/La2O3 for reverse water-gas shift in DBD plasma: Effect of calcination temperature. Energy Conversion and Management. 2020; 206: 112475. doi: 10.1016/j.enconman.2020.112475 DOI: https://doi.org/10.1016/j.enconman.2020.112475
65. Chung KH, Park YK, Kim SJ, et al. CO2-free hydrogen production by liquid-phase plasma cracking from benzene over perovskite catalysts. International Journal of Hydrogen Energy. 2024; 52: 885-893. doi: 10.1016/j.ijhydene.2022.05.008 DOI: https://doi.org/10.1016/j.ijhydene.2022.05.008
66. Alharthi FA, Ababtain AS, Alanazi HS, et al. Synthesis of Zn3V2O8/rGO Nanocomposite for Photocatalytic Hydrogen Production. Inorganics. 2023; 11(3): 93. doi: 10.3390/inorganics11030093 DOI: https://doi.org/10.3390/inorganics11030093
67. Zhang S, Liu D, Song L, et al. Significant improvement of TiO2 photocatalytic hydrogen generation by photothermic synergistic action and underlying mechanism. International Journal of Hydrogen Energy. 2023; 48(69): 26665-26675. doi: 10.1016/j.ijhydene.2023.03.279 DOI: https://doi.org/10.1016/j.ijhydene.2023.03.279
68. He B, Xiao P, Wan S, et al. Rapid charge transfer endowed by interfacial Ni-O bonding in s-scheme heterojunction for efficient photocatalytic H2 and imine production. Angewandte Chemie-International Edition. 2023; 62(50): e202313172. doi: 10.1002/anie.202313172 DOI: https://doi.org/10.1002/anie.202313172
69. Li J, Huang Z, Wang C, et al. Linkage effect in the bandgap-broken V2O5-GdCrO3 heterojunction by carbon allotropes for boosting photocatalytic H2 production. Applied Catalysis B: Environmental. 2024; 340: 123181. doi: 10.1016/j.apcatb.2023.123181 DOI: https://doi.org/10.1016/j.apcatb.2023.123181
70. Li J, Wang X, Fang H, et al. Unraveling the role of surface and interfacial defects in hydrogen production to construct an all-in-one broken-gap photocatalyst. Journal of Materials Chemistry A. 2023; 11(46): 25639-25649. doi: 10.1039/d3ta03079b DOI: https://doi.org/10.1039/D3TA03079B
71. Chelvam K, Hanafiah MM, Woon KS, et al. A review on the environmental performance of various hydrogen production technologies: An approach towards hydrogen economy. Energy Reports. 2024; 11: 369-383. doi: 10.1016/j.egyr.2023.11.060 DOI: https://doi.org/10.1016/j.egyr.2023.11.060
72. Das A, Peu SD. A Comprehensive Review on Recent Advancements in Thermochemical Processes for Clean Hydrogen Production to Decarbonize the Energy Sector. Sustainability. 2022; 14(18): 11206. doi: 10.3390/su141811206 DOI: https://doi.org/10.3390/su141811206
73. Demirbas A. Comparison of thermochemical conversion processes of biomass to hydrogen-rich gas mixtures. Energy Sources, Part A: Recovery, Utilization, and Environmental Effects. 2016; 38(20): 2971-2976. doi: 10.1080/15567036.2015.1122686 DOI: https://doi.org/10.1080/15567036.2015.1122686
74. Preethi V, Kanmani S. Photocatalytic hydrogen production. Materials Science in Semiconductor Processing. 2013; 16(3): 561-575. doi: 10.1016/j.mssp.2013.02.001 DOI: https://doi.org/10.1016/j.mssp.2013.02.001
75. Wang L, Wang S, Zhou J, et al. A scientometric review: Biomass gasification study from 2006 to 2020. ACS Omega. 2022; 7(43): 38246-38253. doi: 10.1021/acsomega.2c05527 DOI: https://doi.org/10.1021/acsomega.2c05527
76. Yan L, He B, Pei X, et al. Design and comparisons of three biomass based hydrogen generation systems with chemical looping process. International Journal of Hydrogen Energy. 2014; 39(31): 17540-17553. doi: 10.1016/j.ijhydene.2014.08.115 DOI: https://doi.org/10.1016/j.ijhydene.2014.08.115
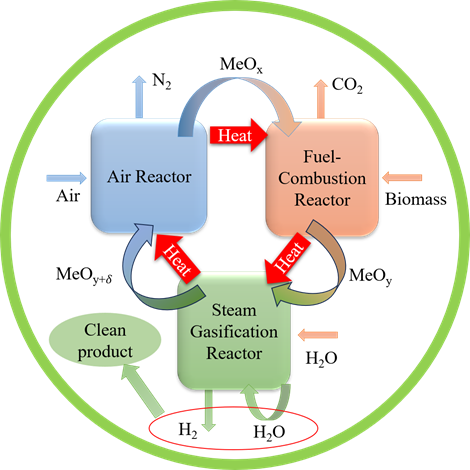
Downloads
Published
How to Cite
Issue
Section
License
Copyright (c) 2024 Yijun Zhang, Yiman Xiao, Siddig Abuelgasim, Chenlong Liu

This work is licensed under a Creative Commons Attribution 4.0 International License.