Screening of high-NMN-producing natural strains and biosynthesis of NMN using Nampt
DOI:
https://doi.org/10.18686/fnc.v1i1.7Keywords:
nicotinamide mononucleotide; fermentation conditions optimization; semi-rational designAbstract
Nicotinamide mononucleotide (NMN) is an endogenous substance in humans with high safety and thermal stability, and its application in cosmetics, medical health, and functional foods has received widespread attention. However, the synthesis process of NMN has problems, such as high cost, time-consuming process, and low yield, which limits the large-scale industrial application of NMN to a certain extent. Nicotinamide phosphoribosyl transferase (Nampt) is a critical enzyme in the technical route of the biological synthesis of NMN, which can catalyze the synthesis of NMN using nicotinamide and phosphoribosyl pyrophosphate. The screening and expression of Nampt with excellent enzymatic properties and stability is the key to the synthesis of NMN via this method. At present, the main problems in the technical route of NMN production using Nampt are that the catalytic activity of Nampt is low and the sources of Nampt are limited. In this study, we isolated Enterobacter chengduensis 2021T4.7, a microorganism with a high NMN production, and optimized its fermentation condition. The yield of NMN was up to 67.66 μM. In addition, we synthesized Nampt and constructed related recombinant high-yield engineered bacteria. We semi-rationally designed a Nampt structure derived from mice and obtained mutant mNampt-V365L with NMN yield as high as 135.99 μM, which increased by 62% from that of the wild type. Here, we screened high-NMN-yield natural strains and obtained high-NMN-yield strains through the semi-rational design optimization of Nampt enzymes, which provided new chassis microorganisms and new ideas for the conversion rate of NMN.
References
1. Wang X, Hu X, Yang Y, et al. Nicotinamide mononucleotide protects against β-amyloid oligomer-induced cognitive impairment and neuronal death. Brain Research 2016; 1643: 1–9. doi: 10.1016/j.brainres.2016.04.060
2. Khan JA, Forouhar F, Tao X, et al. Nicotinamide adenine dinucleotide metabolism as an attractive target for drug discovery. Expert Opinion on Therapeutic Targets 2007; 11(5): 695–705. doi: 10.1517/14728222.11.5.695
3. Fletcher RS, Ratajczak J, Doig CL, et al. Nicotinamide riboside kinases display redundancy in mediating nicotinamide mononucleotide and nicotinamide riboside metabolism in skeletal muscle cells. Molecular Metabolism 2017; 6(8): 819–832. doi: 10.1016/j.molmet.2017.05.011
4. Frederick DW, Loro E, Liu L, et al. Loss of NAD homeostasis leads to progressive and reversible degeneration of skeletal muscle. Cell Metabolism 2016; 24(2): 269–282. doi: 10.1016/j.cmet.2016.07.005
5. Guan Y, Wang SR, Huang XZ, et al. Nicotinamide mononucleotide, an NAD+ precursor, rescues age-associated susceptibility to AKI in a sirtuin 1-dependent manner. Journal of the American Society of Nephrology 2017; 28(8): 2337–2352. doi: 10.1681/asn.2016040385
6. Uddin GM, Youngson NA, Sinclair DA, et al. Head to head comparison of short-term treatment with the NAD+ precursor nicotinamide mononucleotide (NMN) and 6 weeks of exercise in obese female mice. Frontiers in Pharmacology 2016; 7. doi: 10.3389/fphar.2016.00258
7. Fukamizu Y, Uchida Y, Shigekawa A, et al. Safety evaluation of β-nicotinamide mononucleotide oral administration in healthy adult men and women. Scientific Reports 2022; 12(1). doi: 10.1038/s41598-022-18272-y
8. Nadeeshani H, Li J, Ying T, et al. Nicotinamide mononucleotide (NMN) as an anti-aging health product—Promises and safety concerns. Journal of Advanced Research 2022; 37: 267–278. doi: 10.1016/j.jare.2021.08.003
9. Park JH, Long A, Owens K, et al. Nicotinamide mononucleotide inhibits post-ischemic NAD+ degradation and dramatically ameliorates brain damage following global cerebral ischemia. Neurobiology of Disease 2016; 95: 102–110. doi: 10.1016/j.nbd.2016.07.018
10. Yano M, Akazawa H, Yabumoto C, et al. Monocyte–derived extracellular Nampt-dependent biosynthesis of NAD+ protects the heart against pressure overload. Journal of Cardiac Failure 2015; 21(10): S178. doi: 10.1016/j.cardfail.2015.08.188
11. Mills KF, Yoshida S, Stein LR, et al. Long-term administration of nicotinamide mononucleotide mitigates age-associated physiological decline in mice. Cell Metabolism 2016; 24(6): 795–806. doi: 10.1016/j.cmet.2016.09.013
12. Long AN, Owens K, Schlappal AE, et al. Effect of nicotinamide mononucleotide on brain mitochondrial respiratory deficits in an Alzheimer’s disease-relevant murine model. BMC Neurology 2015; 15(1). doi: 10.1186/s12883-015-0272-x
13. Revollo JR, Körner A, Mills KF, et al. Nampt/PBEF/visfatin regulates insulin secretion in β cells as a systemic NAD biosynthetic enzyme. Cell Metabolism 2007; 6(5): 363–375. doi: 10.1016/j.cmet.2007.09.003
14. Caton PW, Kieswich J, Yaqoob MM, et al. Nicotinamide mononucleotide protects against pro-inflammatory cytokine-mediated impairment of mouse islet function. Diabetologia 2011; 54(12): 3083–3092. doi: 10.1007/s00125-011-2288-0
15. Stromsdorfer KL, Yamaguchi S, Yoon MJ, et al. NAMPT–mediated NAD+ biosynthesis in adipocytes regulates adipose tissue function and multi-organ insulin sensitivity in mice. Cell Reports 2016; 16(7): 1851–1860. doi: 10.1016/j.celrep.2016.07.027
16. Marinescu GC, Popescu RG, Stoian G, et al. β-nicotinamide mononucleotide (NMN) production in Escherichia coli. Scientific Reports 2018; 8(1). doi: 10.1038/s41598-018-30792-0
17. Marinescu GC, Popescu RG, Dinischiotu A. Size exclusion chromatography method for purification of nicotinamide mononucleotide (NMN) from bacterial cells. Scientific Reports 2018; 8(1). doi: 10.1038/s41598-018-22806-8
18. Wang P, Xu TY, Guan YF, et al. Perivascular adipose tissue–derived visfatin is a vascular smooth muscle cell growth factor: Role of nicotinamide mononucleotide. Cardiovascular Research 2008; 81(2): 370–380. doi: 10.1093/cvr/cvn288
19. van der Veer E, Ho C, O’Neil C, et al. Extension of human cell lifespan by nicotinamide phosphoribosyltransferase. Journal of Biological Chemistry 2007; 282(15): 10841–10845. doi: 10.1074/jbc.c700018200
20. Burgos ES, Schramm VL. Weak coupling of ATP hydrolysis to the chemical equilibrium of human nicotinamide phosphoribosyltransferase. Biochemistry 2008; 47(42): 11086–11096. doi: 10.1021/bi801198m
21. Cobb RE, Chao R, Zhao H. Directed evolution: Past, present, and future. AIChE Journal 2013; 59(5): 1432–1440. doi: 10.1002/aic.13995
22. Bommarius AS. Biocatalysis: A status report. Annual Review of Chemical and Biomolecular Engineering 2015; 6(1): 319–345. doi: 10.1146/annurev-chembioeng-061114-123415
23. Reetz MT. Biocatalysis in organic chemistry and biotechnology: Past, present, and future. Journal of the American Chemical Society 2013; 135(34): 12480–12496. doi: 10.1021/ja405051f
24. Chen Z, Zeng A. Protein design in systems metabolic engineering for industrial strain development. Biotechnology Journal 2013; 8(5): 523–533. doi: 10.1002/biot.201200238
25. Kipnis Y, Dellus-Gur E, Tawfik DS. TRINS: a method for gene modification by randomized tandem repeat insertions. Protein Engineering Design and Selection 2012; 25(9): 437–444. doi: 10.1093/protein/gzs023
26. Cheng F, Zhu L, Schwaneberg U. Directed evolution 2.0: improving and deciphering enzyme properties. Chemical Communications 2015; 51(48): 9760–9772. doi: 10.1039/c5cc01594d
27. Yu H, Qiu S, Cheng F, et al. Improving the catalytic efficiency of aldo-keto reductase KmAKR towards t-butyl 6-cyano-(3R,5R)-dihydroxyhexanoate via semi-rational design. Bioorganic Chemistry 2019; 90: 103018. doi: 10.1016/j.bioorg.2019.103018
28. Zhang Q, Lu X, Zhang Y, et al. Development of a robust nitrilase by fragment swapping and semi‐rational design for efficient biosynthesis of pregabalin precursor. Biotechnology and Bioengineering 2019; 117(2): 318–329. doi: 10.1002/bit.27203
29. Xu P, Ni ZF, Zong MH, et al. Improving the thermostability and activity of Paenibacillus pasadenensis chitinase through semi-rational design. International Journal of Biological Macromolecules 2020; 150: 9–15. doi: 10.1016/j.ijbiomac.2020.02.033
30. Sugiyama K, Iijima K, Yoshino M, et al. Nicotinamide mononucleotide production by fructophilic lactic acid bacteria. Scientific Reports 2021; 11(1). doi: 10.1038/s41598-021-87361-1
31. Gazzaniga F, Stebbins R, Chang SZ, et al. Microbial NAD metabolism: Lessons from comparative genomics. Microbiology and Molecular Biology Reviews 2009; 73(3): 529–541. doi: 10.1128/mmbr.00042-08
32. Malakhov MP, Mattern MR, Malakhova OA, et al. SUMO fusions and SUMO-specific protease for efficient expression and purification of proteins. Journal of Structural and Functional Genomics 2004; 5(1–2): 75–86. doi: 10.1023/B:JSFG.0000029237.70316.52
33. Johnson ES. Protein modification by SUMO. Annual Review of Biochemistry 2004; 73(1): 355–382. doi: 10.1146/annurev.biochem.73.011303.074118
34. Lutz S. Beyond directed evolution–semi-rational protein engineering and design. Current Opinion in Biotechnology 2010; 21(6): 734–743. doi: 10.1016/j.copbio.2010.08.011
35. Liao YB, Wu MH, Ling SL, et al. Expression of nicotinamide phosphoribosyltransferase in Escherichia coli and catalytic synthesis of nicotinamide mononucleotide. Modern Food Science and Technology 2021; 37(2): 87–93, 182.
36. Reetz MT, Carballeira JD. Iterative saturation mutagenesis (ISM) for rapid directed evolution of functional enzymes. Nature Protocols 2007; 2(4): 891–903. doi: 10.1038/nprot.2007.72
37. Imai S, Yoshino J. The importance of Nampt/NAD/SIRT1 in the systemic regulation of metabolism and ageing. Diabetes, Obesity and Metabolism 2013; 15(s3): 26–33. doi: 10.1111/dom.12171
38. He Z, Yang X, Tian X, et al. Yeast cell surface engineering of a nicotinamide riboside kinase for the production of β–nicotinamide mononucleotide via whole–Cell catalysis. ACS Synthetic Biology 2022; 11(10): 3451–3459. doi: 10.1021/acssynbio.2c00350
39. Huang Z, Li N, Yu S, et al. Systematic engineering of Escherichia coli for efficient production of nicotinamide mononucleotide from nicotinamide. ACS Synthetic Biology 2022; 11(9): 2979–2988. doi: 10.1021/acssynbio.2c00100
40. Kurnasov OV, Polanuyer BM, Ananta S, et al. Ribosylnicotinamide kinase domain of NadR protein: Identification and implications in NAD+ biosynthesis. Journal of Bacteriology 2002; 184(24): 6906–6917. doi: 10.1128/jb.184.24.6906-6917.2002
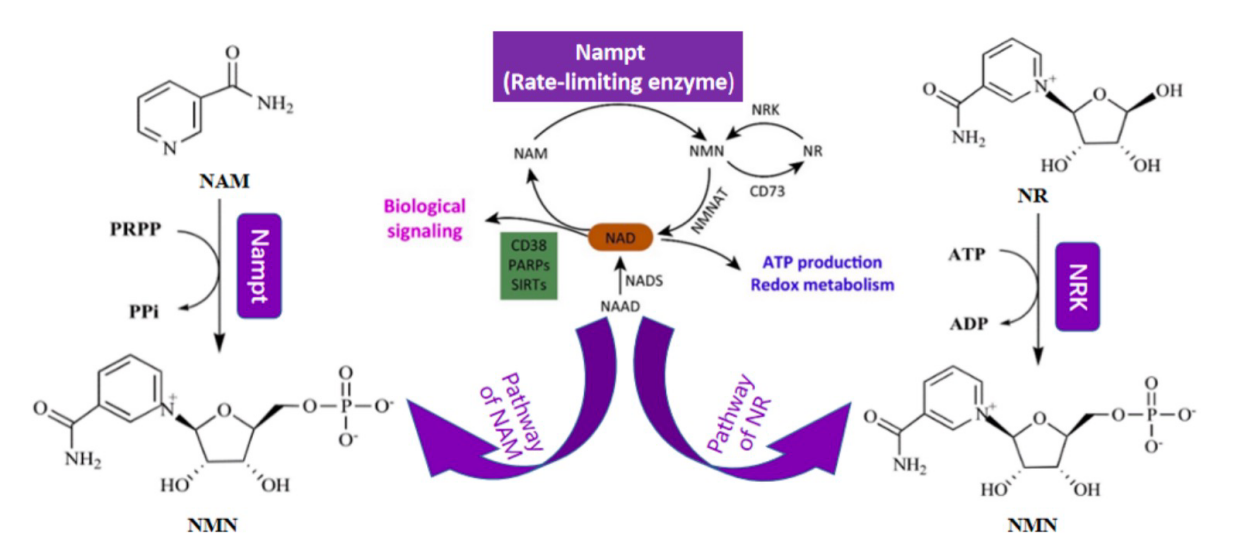
Downloads
Published
How to Cite
Issue
Section
License
Copyright (c) 2023 Chao Li, Zouguo Long, Haichao Zhang, Yuhui Lin, Liqing Zhao

This work is licensed under a Creative Commons Attribution-NonCommercial 4.0 International License.