超临界水热燃烧:基本原理、特性规律及其在能源环境领域中应用与开发
DOI:
https://doi.org/10.18686/cncest.v1i2.93关键词:
超临界水热燃烧;燃烧特性;数值模拟;废物处理;煤炭地下气化;稠油热采摘要
超临界水热燃烧技术是一种“水火相容”、无NOx与SOx生成的新型清洁均相燃烧技术,有望推动有机废物高效处理、传统化石能源清洁利用、稠油高效热采等领域技术的变革性发展。本文在归纳近年来超临界水热燃烧技术基本特点的基础上,重点从实验研究、数值计算两个角度总结分析了典型燃料的超临界水热燃烧特性与规律,以及在能源环境领域变革性工程应用与开发的最新进展。指出降低着火温度与熄火温度是推进超临界水热燃烧技术应用的关键,考虑真实流体效应与湍流-反应交互作用才能正确反映燃烧过程。此外,超临界水热燃烧作为体系热量与反应介质供给来源,可实现高浓有机废物高效去除、煤炭清洁燃烧与原位制氢以及生成多元热流体强化稠油热采。当前,超临界水热燃烧强迫点火技术、反应器设计准则、关键设备防腐仍是未来研究的重点,对推进超临界水热燃烧技术工程化成熟具有重要意义。
参考
National Bureau of Statistics. Statistical bulletin of the national economic and social development of the People’s
Republic of China in 2022 (Chinese). China Statistics 2023; 3: 12–29.
Liu S, Chang Z, Liu J. Key technologies and prospect for in-situ gasification mining of deep coal resources.
Journal of Mining Science and Technology 2021; 6(3): 261–270. doi: 10.19606/j.cnki.jmst.2021.03.002
Huang S, Cao M, Cheng L. Experimental study on the mechanism of enhanced oil recovery by multi-thermal fluid
in offshore heavy oil. International Journal of Heat and Mass Transfer 2018; 122: 1074–1084. doi:
1016/j.ijheatmasstransfer.2018.02.049
Schilling W, Franck EU. Combustion and diffusion flames at high pressures to 2000 bar. Berichte der
Bunsengesellschaft für physikalische Chemie 1988; 92(5): 631–636. doi: 10.1002/bbpc.198800149
Steeper RR, Rice SF, Brown MS, et al. Methane and methanol diffusion flames in supercritical water. The Journal
of Supercritical Fluids 1992; 5(4): 262–268. doi: 10.1016/0896-8446(92)90017-e
Sobhy A, Guthrie RIL, Butler IS, et al. Naphthalene combustion in supercritical water flames. Proceedings of the
Combustion Institute 2009; 32(2): 3231–3238. doi: 10.1016/j.proci.2008.06.173
Meier T, Schuler MJ, Stathopoulos P, et al. Hot surface ignition and monitoring of an internal oxygen–ethanol
hydrothermal flame at 260 bar. The Journal of Supercritical Fluids 2017; 130: 230–238. doi:
1016/j.supflu.2016.09.015
Wellig B, Weber M, Lieball K, et al. Hydrothermal methanol diffusion flame as internal heat source in a SCWO
reactor. The Journal of Supercritical Fluids 2009; 49(1): 59–70. doi: 10.1016/j.supflu.2008.11.021
Stathopoulos P, Ninck K, von Rohr PR. Hot-wire ignition of ethanol–oxygen hydrothermal flames. Combustion
and Flame 2013; 160(11): 2386–2395. doi: 10.1016/j.combustflame.2013.05.006
Bermejo MD, Cabeza P, Queiroz JPS, et al. Analysis of the scale up of a transpiring wall reactor with a
hydrothermal flame as a heat source for the supercritical water oxidation. The Journal of Supercritical Fluids
; 56(1): 21–32. doi: 10.1016/j.supflu.2010.11.014
Bermejo MD, Jiménez C, Cabeza P, et al. Experimental study of hydrothermal flames formation using a tubular
injector in a refrigerated reaction chamber. Influence of the operational and geometrical parameters. The Journal
of Supercritical Fluids 2011; 59: 140–148. doi: 10.1016/j.supflu.2011.08.009
Cabeza P, Silva Queiroz JP, Criado M, et al. Supercritical water oxidation for energy production by hydrothermal
flame as internal heat source. Experimental results and energetic study. Energy 2015; 90: 1584–1594. doi:
1016/j.energy.2015.06.118
Hicks MC, Hegde UG, Kojima JJ. Hydrothermal ethanol flames in Co-flow jets. The Journal of Supercritical
Fluids 2019; 145: 192–200. doi: 10.1016/j.supflu.2018.12.010
Xu D, Wang S, Huang C, et al. Transpiring wall reactor in supercritical water oxidation. Chemical Engineering
Research and Design 2014; 92(11): 2626–2639. doi: 10.1016/j.cherd.2014.02.028
Cui C, Li Y, Wang S, et al. Review on an advanced combustion technology: Supercritical hydrothermal
combustion. Applied Sciences 2020; 10(5): 1645. doi: 10.3390/app10051645
清洁能源科学与技术 第 1 卷 第 2 期(2023) 25/28
Shaw RW. Supercritical water a medium for chemistry. Chemical Engineering News 1991; 69(51): 26–39. doi:
1021/cen-v069n051.p026
Huang G. Properties of water in supercritical state (Chinese). Northwest China Electric Power 2004; 4: 82–84, 86.
Liu X, Guo Y, Lin L. Application of super (sub)critical water in chemical reactions (Chinese). Inner Mongolia
Petrochemical Industry 2008; 3: 34–37.
Tian Y, Feng J, Qin Y, et al. Properties of supercritical water and its application in chemical reactions (Chinese).
Chemistry (Huaxue Tongbao) 2002; 6: 396–402.
YangK, Xu M, Lin C. Physical and chemical properties of supercritical water (Chinese). Journal of Zhejiang
University of Technology 2001; 4: 66–70.
Zhang L, Chen L, Zhao X, et al. Properties of supercritical water and its application. Chemical Industry and
Engineering 2003; 20(1): 33–38, 54.
Augustine C, Tester JW. Hydrothermal flames: From phenomenological experimental demonstrations to
quantitative understanding. The Journal of Supercritical Fluids 2009; 47(3): 415–430. doi:
1016/j.supflu.2008.10.003
Reddy SN, Nanda S, Hegde UG, et al. Ignition of hydrothermal flames. RSC Advances 2015; 5(46): 36404–36422.
doi: 10.1039/c5ra02705e
Jiang Z, Li Y, Wang S, et al. Review on mechanisms and kinetics for supercritical water oxidation processes.
Applied Sciences 2020; 10(14): 4937. doi: 10.3390/app10144937
Henrikson JT, Grice CR, Savage PE. Effect of water density on methanol oxidation kinetics in supercritical water.
The Journal of Physical Chemistry A 2006; 110(10): 3627–3632. doi: 10.1021/jp057184u
Richard Holgate H, Tester JW. Fundamental kinetics and mechanisms of hydrogen oxidation in supercritical
water. Combustion Science and Technology 1993; 88(5–6): 369–397. doi: 10.1080/00102209308947245
Fujii T, Hayashi R, Kawasaki S, et al. Water density effects on methanol oxidation in supercritical water at high
pressure up to 100 MPa. The Journal of Supercritical Fluids 2011; 58(1): 142–149. doi:
1016/j.supflu.2011.04.004
Boock LT, Klein MT. Lumping strategy for modeling the oxidation of C1–C3 alcohols and acetic acid in hightemperature water. Industrial & Engineering Chemistry Research 1993; 32(11): 2464–2473. doi:
1021/ie00023a004
Cabeza P, Bermejo MD, Jiménez C, et al. Experimental study of the supercritical water oxidation of recalcitrant
compounds under hydrothermal flames using tubular reactors. Water Research 2011; 45(8): 2485–2495. doi:
1016/j.watres.2011.01.029
Zhang J, Wang S, Xu D, et al. Kinetics study on hydrothermal combustion of methanol in supercritical water.
Chemical Engineering Research and Design 2015; 98: 220–230. doi: 10.1016/j.cherd.2015.05.002
Zhang F, Zhang Y, Xu C, et al. Experimental study on the ignition and extinction characteristics of the
hydrothermal flame. Chemical Engineering & Technology 2015; 38(11): 2054–2066. doi: 10.1002/ceat.201300571
Bermejo MD, Cabeza P, Bahr M, et al. Experimental study of hydrothermal flames initiation using different static
mixer configurations. The Journal of Supercritical Fluids 2009; 50(3): 240–249. doi:
1016/j.supflu.2009.06.010
Sobhy A, Butler IS, Kozinski JA. Selected profiles of high-pressure methanol–air flames in supercritical water.
Proceedings of the Combustion Institute 2007; 31(2): 3369–3376. doi: 10.1016/j.proci.2006.07.253
Reddy SN, Nanda S, Kumar P, et al. Impacts of oxidant characteristics on the ignition of n-propanol-air
hydrothermal flames in supercritical water. Combustion and Flame 2019; 203: 46–55. doi:
1016/j.combustflame.2019.02.004
Hirth Th, Franck EU. Oxidation and hydrothermolysis of hydrocarbons in supercritical water at high pressures.
Berichte der Bunsengesellschaft für physikalische Chemie 1993; 97(9): 1091–1097. doi:
1002/bbpc.19930970905
Savage PE. Organic chemical reactions in supercritical water. Chemical Reviews 1999; 99(2): 603–622. doi:
1021/cr9700989
Meier T, Stathopoulos P, von Rohr PR. Hot surface ignition of oxygen–ethanol hydrothermal flames. The Journal
of Supercritical Fluids 2016; 107: 462–468. doi: 10.1016/j.supflu.2014.11.012
Wellig B, Lieball K, von Rohr Rudolf. Operating characteristics of a transpiring-wall SCWO reactor with a
hydrothermal flame as internal heat source. Journal of Supercritical Fluids 2005; 34(1): 35–50. doi:
1016/j.supflu.2004.07.003
Zhang F, Chen S, Xu C, et al. Experimental study on the effects of operating parameters on the performance of a
transpiring-wall supercritical water oxidation reactor. Desalination 2012; 294: 60–66. doi:
1016/j.desal.2012.03.013
Zhang F, Xu C, Zhang Y, et al. Experimental study on the operating characteristics of an inner preheating
transpiring wall reactor for supercritical water oxidation: Temperature profiles and product properties. Energy
; 66: 577–587. doi: 10.1016/j.energy.2014.02.003
清洁能源科学与技术 第 1 卷 第 2 期(2023) 26/28
Bermejo MD, Fdez-Polanco F, Cocero MJ. Experimental study of the operational parameters of a transpiring wall
reactor for supercritical water oxidation. The Journal of Supercritical Fluids 2006; 39(1): 70–79. doi:
1016/j.supflu.2006.02.006
Serikawa R. Hydrothermal flames in supercritical water oxidation: Investigation in a pilot scale continuous
reactor. Fuel 2002; 81(9): 1147–1159. doi: 10.1016/s0016-2361(02)00015-7
Cabeza P, Queiroz JPS, Arca S, et al. Sludge destruction by means of a hydrothermal flame. Optimization of
ammonia destruction conditions. Chemical Engineering Journal 2013; 232: 1–9. doi: 10.1016/j.cej.2013.07.040
Príkopský K. Characterization of Continuous Diffusion Flames in Supercritical Water [PhD thesis]. ETH Zurich;
Schanzenbächer J, Taylor JD, Tester JW. Ethanol oxidation and hydrolysis rates in supercritical water. The
Journal of Supercritical Fluids 2002; 22(2): 139–147. doi: 10.1016/s0896-8446(01)00119-x
Vogel F, Blanchard JLD, Marrone PA, et al. Critical review of kinetic data for the oxidation of methanol in
supercritical water. The Journal of Supercritical Fluids 2005; 34(3): 249–286. doi: 10.1016/j.supflu.2003.12.018
Ren M. Research on Supercritical Hydrothermal Combustion Mechanism Using Reaction Kinetics Coupled Flow
Model [PhD thesis]. Xi’an Jiaotong University; 2019.
Brock EE, Savage PE. Detailed chemical kinetics model for supercritical water oxidation of C1 compounds and
H2. AIChE Journal 1995; 41(8): 1874–1888. doi: 10.1002/aic.690410806
Baulch DL, Cobos CJ, Cox RA, et al. Evaluated kinetic data for combustion modelling. Journal of Physical and
Chemical Reference Data 1992; 21(3): 411. doi: 10.1063/1.555908
Baulch DL, Cobos CJ, Cox RA, et al. Evaluated kinetic data for combustion modeling. Supplement I. Journal of
Physical and Chemical Reference Data 1994; 23(6): 847–848. doi: 10.1063/1.555953
Rice SF, Croiset E. Oxidation of simple alcohols in supercritical water III. Formation of intermediates from
ethanol. Industrial & Engineering Chemistry Research 2000; 40(1): 86–93. doi: 10.1021/ie000372g
Marinov NM. A detailed chemical kinetic model for high temperature ethanol oxidation. International Journal of
Chemical Kinetics 1999; 31(3): 183–220. doi: 10.1002/(SICI)1097-4601(1999)31:3<183::AID-KIN3>3.0.CO;2-X
Ploeger JM, Green WH, Tester JW. Co-oxidation of methylphosphonic acid and ethanol in supercritical water. The
Journal of Supercritical Fluids 2006; 39(2): 239–245. doi: 10.1016/j.supflu.2006.03.003
Ren M, Wang S, Zhang J, et al. Characteristics of methanol hydrothermal combustion: Detailed chemical kinetics
coupled with simple flow modeling study. Industrial & Engineering Chemistry Research 2017; 56(18): 5469–
doi: 10.1021/acs.iecr.7b00886
Li L, Chen P, Gloyna EF. Generalized kinetic model for wet oxidation of organic compounds. AIChE Journal
; 37(11): 1687–1697. doi: 10.1002/aic.690371112
Hayashi R, Onishi M, Sugiyama M, et al. Kinetic analysis on alcohol concentration and mixture effect in
supercritical water oxidation of methanol and ethanol by elementary reaction model. The Journal of Supercritical
Fluids 2007; 40(1): 74–83. doi: 10.1016/j.supflu.2006.04.006
Ploeger JM, Green WH, Tester JW. Co‐oxidation of ammonia and ethanol in supercritical water, part 2: Modeling
demonstrates the importance of H2NNOx. International Journal of Chemical Kinetics 2008; 40(10): 653–662. doi:
1002/kin.20345
Benjamin KM, Savage PE. Detailed chemical kinetic modeling of methylamine in supercritical water. Industrial &
Engineering Chemistry Research 2005; 44(26): 9785–9793. doi: 10.1021/ie050926l
Dinaro JL, Howard JB, Green WH, et al. Analysis of an elementary reaction mechanism for benzene oxidation in
supercritical water. Proceedings of the Combustion Institute 2000; 28(2): 1529–1536. doi: 10.1016/s0082-
(00)80550-0
Gao Z, Wang H, Song C, et al. Real-fluid effects on laminar diffusion and premixed hydrothermal flames. The
Journal of Supercritical Fluids 2019; 153: 104566. doi: 10.1016/j.supflu.2019.104566
Chung TH, Ajlan M, Lee LL, et al. Generalized multiparameter correlation for nonpolar and polar fluid transport
properties. Industrial & Engineering Chemistry Research 1988; 27(4): 671–679. doi: 10.1021/ie00076a024
Narayanan C, Frouzakis C, Boulouchos K, et al. Numerical modelling of a supercritical water oxidation reactor
containing a hydrothermal flame. The Journal of Supercritical Fluids 2008; 46(2): 149–155. doi:
1016/j.supflu.2008.04.005
Sierra-Pallares J, Teresa Parra-Santos M, García-Serna J, et al. Numerical modelling of hydrothermal flames.
Micromixing effects over turbulent reaction rates. The Journal of Supercritical Fluids 2009; 50(2): 146–154. doi:
1016/j.supflu.2009.05.001
Poling BE, Prausnitz JM, O’Connell JP. The Properties of Gases and Liquids, 5th ed. McGraw-Hill; 2001.
Hjertager LK, Hjertager BH, Solberg T. CFD modelling of fast chemical reactions in turbulent liquid flows.
Computers & Chemical Engineering 2002; 26(4–5): 507–515. doi: 10.1016/s0098-1354(01)00799-2
Mathur GP, Thodos G. The self‐diffusivity of substances in the gaseous and liquid states. AIChE Journal 1965;
(4): 613–616. doi: 10.1002/aic.690110411
清洁能源科学与技术 第 1 卷 第 2 期(2023) 27/28
Zhang J, Ren M, Li Y, et al. Continuous supercritical hydrothermal combustion experimental and burner structure
optimization simulation study. Chemical Engineering Research and Design 2022; 188: 69–80. doi:
1016/j.cherd.2022.09.039
Song C. Direct Numerical Simulation Study of Supercritical Hydrothermal Combustion (Chiense) [PhD thesis].
Zhejiang University; 2020.
Qian L, Wang S, Ren M, et al. Co-oxidation effects and mechanisms between sludge and alcohols (methanol,
ethanol and isopropanol) in supercritical water. Chemical Engineering Journal 2019; 366: 223–234. doi:
1016/j.cej.2019.02.046
Ren M, Wang S, Yang C, et al. Supercritical water oxidation of quinoline with moderate preheat temperature and
initial concentration. Fuel 2019; 236: 1408–1414. doi: 10.1016/j.fuel.2018.09.091
Zhang J, Li P, Lu J, et al. Supercritical water oxidation of ammonia with methanol as the auxiliary fuel:
Comparing with isopropanol. Chemical Engineering Research and Design 2019; 147: 160–170. doi:
1016/j.cherd.2019.05.010
Zhang J, Li P, Lu J, et al. Supercritical hydrothermal combustion of nitrogen-containing compounds in a tubular
reactor. Fuel 2020; 275: 117889. doi: 10.1016/j.fuel.2020.117889
Fujie M, Inui A, Anjo KJ, et al. Study of combustion behavior of brown coal in supercritical water. High Pressure
Research 2001; 20(1–6): 385–392. doi: 10.1080/08957950108206186
Ma H. Research on Basic Issues of Coal Supercritical Water Gasification Coupled Hydrothermal Combustion
Power Generation System (Chiense) [PhD thesis]. Xi’an Jiaotong University; 2013.
Bermejo MD, Cocero MJ, Fernández-Polanco F. A process for generating power from the oxidation of coal in
supercritical water. Fuel 2004; 83(2): 195–204. doi: 10.1016/s0016-2361(03)00256-4
Liang W. Research on the Hydrothermal Combustion of Coal in Supercritical Water [Master’s thesis]. Xi’an
Jiaotong University; 2007.
Chen J, Wang L, Cheng Z, et al. Performance simulation and thermodynamics analysis of hydrogen production
based on supercritical water gasification of coal. International Journal of Hydrogen Energy 2021; 46(56): 28474–
doi: 10.1016/j.ijhydene.2021.06.097
Zhang F, Wang S, Duan Y, et al. Thermodynamic assessment of hydrothermal combustion assisted fossil fuel insitu gasification in the context of sustainable development. Fuel 2023; 335: 127053. doi:
1016/j.fuel.2022.127053
Liu W, Wan Y, Xiong Y, et al. Green hydrogen standard in China: Standard and evaluation of low-carbon
hydrogen, clean hydrogen, and renewable hydrogen. International Journal of Hydrogen Energy 2022; 47(58):
–24591. doi: 10.1016/j.ijhydene.2021.10.193
Guo Y. Research progress of viscosity reduction of heavy oil. Contemporary Chemical Industry 2019; 48(1): 4.
He W, Li Z, Li Y, et al. Prospects of supercritical hydrothermal combustion as recovery technology for heavy oil
reservoirs. Geoenergy Science and Engineering 2023; 227: 211795. doi: 10.1016/j.geoen.2023.211795
Wang S, Xu H, Li Y, et al. A Supercritical Hydrothermal Combustion Steam Generator for Ultra-Deep
Underground Heavy Oil Thermal Recovery (Chinese). China Patent 110617466A, 27 December 2019.
Wang S, Cui C, Li Y, et al. A Supercritical Hydrothermal Combustion Device Suitable for High-Viscosity Fuels
(Chinese). China Patent CN110645555A, 3 January 2020.
Geng Y, Wang S, Zhang F, et al. Numerical investigations on hydrothermal flame characteristics of water-cooled
hydrothermal burner. International Journal of Chemical Reactor Engineering 2023; 21(10): 1225–1239. doi:
1515/ijcre-2023-0040
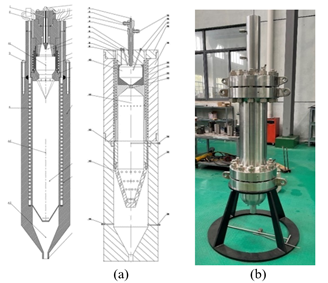
##submission.downloads##
已出版
文章引用
期
栏目
执照
版权声明
CC BY-NC 4.0