含有纳米碳填料的3D打印可拉伸导电聚合物复合材料的多功能应用
DOI:
https://doi.org/10.18686/cncest.v1i2.91关键词:
碳基材料;3D打印技术;聚合物复合材料;结构设计;电子设备摘要
碳纳米材料因其稳定性好、导电性强、价格低廉而被广泛用作基底材料来制备可拉伸导电复合材料。针对优化复合材料性能的需求,各种制备碳纳米材料增强可拉伸导电复合材料的制造方法应运而生。其中,3D打印技术具有工艺灵活、产品性能优良等优点,受到广泛关注。本文重点综述利用3D打印技术在高分子材料中添加碳纳米材料作为增强相的研究进展。 展望了基于纳米碳填料的导电聚合物复合材料在航空航天、储能、生物医学等领域的应用前景。
参考
1. Cao D, Xing Y, Tantratian K, et al. 3D printed high-performance lithium metal microbatteries enabled by nanocellulose. Advanced Materials 2019; 31(14): 1807313. doi: 10.1002/adma.201807313
2. Chen X. Making electrodes stretchable. Small Methods 2017; 1(4): 1600029. doi: 10.1002/smtd.201600029
3. Lv Z, Li W, Yang L, et al. Custom-made electrochemical energy storage devices. ACS Energy Letters 2019; 4(2): 606–614. doi: 10.1021/acsenergylett.8b02408
4. Zhao C, Wang R, Fang B, et al. Boosting the lithium storage properties of a flexible Li4Ti5O12/graphene fiber anode via a 3D printing assembly strategy. Batteries 2023; 9(10): 493. doi: 10.3390/batteries9100493
5. Wang C, Xia K, Wang H, et al. Advanced carbon for flexible and wearable electronics. Advanced Materials 2019; 31(9): 1801072. doi: 10.1002/adma.201801072
6. Bokobza L. Mechanical and electrical properties of elastomer nanocomposites based on different carbon nanomaterials. C—Journal of Carbon Research 2017; 3(2): 10. doi: 10.3390/c3020010
7. Mondal S, Khastgir D. Elastomer reinforcement by graphene nanoplatelets and synergistic improvements of electrical and mechanical properties of composites by hybrid nano fillers of graphene-carbon black & graphene-MWCNT. Composites Part A: Applied Science and Manufacturing 2017; 102: 154–165. doi: 10.1016/j.compositesa.2017.08.003
8. Ryan KR, Down MP, Hurst NJ, et al. Additive manufacturing (3D printing) of electrically conductive polymers and polymer nanocomposites and their applications. eScience 2022; 2(4): 365–381. doi: 10.1016/j.esci.2022.07.003
9. Huang A, Ma Y, Peng J, et al. Tailoring the structure of silicon-based materials for lithium-ion batteries via electrospinning technology. eScience 2021; 1(2): 141–162. doi: 10.1016/j.esci.2021.11.006
10. Wang Z, Gao W, Zhang Q, et al. 3D-printed graphene/polydimethylsiloxane composites for stretchable and strain-insensitive temperature sensors. ACS Applied Materials & Interfaces 2019; 11(1): 1344–1352. doi: 10.1021/acsami.8b16139
11. Mo R, Rooney D, Sun K, Yang HY. 3D nitrogen-doped graphene foam with encapsulated germanium/nitrogen-doped graphene yolk-shell nanoarchitecture for high-performance flexible Li-ion battery. Nature Communications 2017; 8(1): 13949. doi: 10.1038/ncomms13949
12. Zhao C, Liang H, Wang R, et al. Recent advances in high value-added carbon materials prepared from carbon dioxide for energy storage applications. Carbon Capture Science & Technology 2023; 9: 100144. doi: 10.1016/j.ccst.2023.100144
13. de Leon AC, Chen Q, Palaganas NB, et al. High performance polymer nanocomposites for additive manufacturing applications. Reactive and Functional Polymers 2016; 103: 141–155. doi: 10.1016/j.reactfunctpolym.2016.04.010
14. Song WJ, Lee S, Song G, Park S. Stretchable aqueous batteries: Progress and prospects. ACS Energy Letters 2019; 4(1): 177–186. doi: 10.1021/acsenergylett.8b02053
15. Song Z, Ma T, Tang R, et al. Origami lithium-ion batteries. Nature Communications 2014; 5(1): 3140. doi: 10.1038/ncomms4140
16. Bao Y, Zhang XY, Zhang X, et al. Free-standing and flexible limntio4/carbon nanotube cathodes for high performance lithium ion batteries. Journal of Power Sources 2016; 321: 120–125. doi: 10.1016/j.jpowsour.2016.04.121
17. Fu KK, Cheng J, Li T, Hu L. Flexible batteries: From mechanics to devices. ACS Energy Letters 2016; 1(5): 1065–1079. doi: 10.1021/acsenergylett.6b00401
18. Bao Y, Hong G, Chen Y, et al. Customized kirigami electrodes for flexible and deformable lithium-ion batteries. ACS Applied Materials & Interfaces 2020; 12(1): 780–788. doi: 10.1021/acsami.9b18232
19. Storck JL, Ehrmann G, Uthoff J, Diestelhorst E. Investigating inexpensive polymeric 3D printed materials under extreme thermal conditions. Materials Futures 2022; 1(1): 015001. doi: 10.1088/2752-5724/ac4beb
20. de Castro Motta J, Qaderi S, Farina I, et al. Experimental characterization and mechanical modeling of additively manufactured TPU components of innovative seismic isolators. Acta Mechanica 2022. doi: 10.1007/s00707-022-03447-5
21. Buchanan C, Gardner L. Metal 3D printing in construction: A review of methods, research, applications, opportunities and challenges. Engineering Structures 2019; 180: 332–348. doi: 10.1016/j.engstruct.2018.11.045
22. Zhu C, Liu T, Qian F, et al. 3D printed functional nanomaterials for electrochemical energy storage. Nano Today 2017; 15: 107–120. doi: 10.1016/j.nantod.2017.06.007
23. Zhang F, Wei M, Viswanathan VV, et al. 3D printing technologies for electrochemical energy storage. Nano Energy 2017; 40: 418–431. doi: 10.1016/j.nanoen.2017.08.037
24. Sousa RE, Costa CM, Lanceros-Méndez S. Advances and future challenges in printed batteries. ChemSusChem 2015; 8(21): 3539–3555. doi: 10.1002/cssc.201500657
25. Tian X, Jin J, Yuan S, et al. Emerging 3D-printed electrochemical energy storage devices: A critical review. Advanced Energy Materials 2017; 7(17): 1700127. doi: 10.1002/aenm.201700127
26. Guo W, Wang X, Yang C, et al. Microfluidic 3D printing polyhydroxyalkanoates-based bionic skin for wound healing. Materials Futures 2022; 1: 015401. doi: 10.1088/2752-5724/ac446b
27. Park S, Shou W, Makatura L, et al. 3D printing of polymer composites: Materials, processes, and applications. Matter 2022; 5(1): 43–76. doi: 10.1016/j.matt.2021.10.018
28. de Leon AC, Rodier BJ, Bajamundi C, et al. Plastic metal-free electric motor by 3D printing of graphene-polyamide powder. ACS Applied Energy Materials 2018; 1(4): 1726–1733. doi: 10.1021/acsaem.8b00240
29. Hall A, Kong GX, Karanassios V. Detectors and light-sources for optical spectrometry: From a 3D-printed light-source to a self-powered sensor fabricated on a flexible polymeric substrate, and from there on to an IoT-enabled “smart” system. In: Proceedings of the 2019 IEEE International Conference on Flexible and Printable Sensors and Systems (FLEPS); 8–10 July 2019; Glasgow, UK. pp. 1–3. doi:10.1109/fleps.2019.8792321
30. Kurra N, Jiang Q, Nayak P, Alshareef HN. Laser-derived graphene: A three-dimensional printed graphene electrode and its emerging applications. Nano Today 2019; 24: 81–102. doi: 10.1016/j.nantod.2018.12.003
31. Li C, Cheng J, He Y, et al. Polyelectrolyte elastomer-based ionotronic sensors with multi-mode sensing capabilities via multi-material 3D printing. Nature Communications 2023; 14(1): 4853. doi: 10.1038/s41467-023-40583-5
32. Li K, Liang M, Wang H, et al. 3D mxene architectures for efficient energy storage and conversion. Advanced Functional Materials 2020; 30(47): 2000842. doi: 10.1002/adfm.202000842
33. Lyu Z, Lim GJH, Koh JJ, et al. Design and manufacture of 3D-printed batteries. Joule 2021; 5(1): 89–114. doi: 10.1016/j.joule.2020.11.010
34. Park J, Kim JK, Park SA, et al. 3D-printed biodegradable polymeric stent integrated with a battery-less pressure sensor for biomedical applications. In: Proceedings of the 2017 19th International Conference on Solid-State Sensors, Actuators and Microsystems (TRANSDUCERS); 18–22 June 2017; Kaohsiung, Taiwan. pp. 14–50. doi: 10.1109/transducers.2017.7993984
35. Ngo TD, Kashani A, Imbalzano G, et al. Additive manufacturing (3D printing): A review of materials, methods, applications and challenges. Composites Part B: Engineering 2018; 143: 172–196. doi: 10.1016/j.compositesb.2018.02.012
36. Zhu Y, Murali S, Cai W, et al. Graphene and graphene oxide: Synthesis, properties, and applications. Advanced Materials 2010; 22(35): 3906–3024. doi: 10.1002/adma.201001068
37. Unwin PR, Güell AG, Zhang G. Nanoscale electrochemistry of sp2 carbon materials: From graphite and graphene to carbon nanotubes. Accounts of Chemical Research 2016; 49(9): 2041–2408. doi: 10.1021/acs.accounts.6b00301
38. Smith M. New developments in carbon fiber. Reinforced Plastics 2018; 62(5): 266–269. doi: 10.1016/j.repl.2017.07.004
39. Fu X, Xu L, Li J, et al. Flexible solar cells based on carbon nanomaterials. Carbon 2018; 139: 1063–1073. doi: 10.1016/j.carbon.2018.08.017
40. Bhagavatheswaran ES, Parsekar M, Das A, et al. Construction of an interconnected nanostructured carbon black network: Development of highly stretchable and robust elastomeric conductors. The Journal of Physical Chemistry C 2015; 119(37): 21723–21731. doi: 10.1021/acs.jpcc.5b06629
41. Niu XZ, Peng SL, Liu LY, et al. Characterizing and patterning of PDMS-based conducting composites. Advanced Materials 2007; 19(18): 2682–2686. doi: 10.1002/adma.200602515
42. Song WJ, Park J, Kim DH, et al. Jabuticaba-inspired hybrid carbon filler/polymer electrode for use in highly stretchable aqueous Li-ion batteries. Advanced Energy Materials 2018; 8(10): 1702478. doi: 10.1002/aenm.201702478
43. Shin MK, Oh J, Lima M, et al. Elastomeric conductive composites based on carbon nanotube forests. Advanced Materials 2010; 22(24): 2663–2667. doi: 10.1002/adma.200904270
44. Sekitani T, Nakajima H, Maeda H, et al. Stretchable active-matrix organic light-emitting diode display using printable elastic conductors. Nature Materials 2009; 8(6): 494–499. doi: 10.1038/nmat2459
45. Liu Z, Qian Z, Song J, Zhang Y. Conducting and stretchable composites using sandwiched graphene-carbon nanotube hybrids and styrene-butadiene rubber. Carbon 2019; 149: 181–189. doi: 10.1016/j.carbon.2019.04.037
46. Gao N, Fang X. Synthesis and development of graphene—Inorganic semiconductor nanocomposites. Chemical Reviews 2015; 115(16): 8294–83343. doi: 10.1021/cr400607y
47. Chen Z, Ren W, Gao L, et al. Three-dimensional flexible and conductive interconnected graphene networks grown by chemical vapour deposition. Nature Materials 2011; 10(6): 424–428. doi: 10.1038/nmat3001
48. Wang Z, Liu X, Shen X, et al. An ultralight graphene honeycomb sandwich for stretchable light-emitting displays. Advanced Functional Materials 2018; 28(19): 1707043. doi: 10.1002/adfm.201707043
49. Sun F, Tian M, Sun X, et al. Stretchable conductive fibers of ultrahigh tensile strain and stable conductance enabled by a worm-shaped graphene microlayer. Nano Letters 2019; 19(9): 6592–6599. doi: 10.1021/acs.nanolett.9b02862
50. Praveena BA, Lokesh N, Buradi A, et al. A comprehensive review of emerging additive manufacturing (3D printing technology): Methods, materials, applications, challenges, trends and future potential. Materials Today: Proceedings 2022; 52(Part 3): 1309–1313. doi: 10.1016/J.MATPR.2021.11.059
51. Kristiawan RB, Imaduddin F, Ariawan D, et al. A review on the fused deposition modeling (FDM) 3D printing: Filament processing, materials, and printing parameters. Open Engineering 2021; 11(1): 639–649. doi: 10.1515/ENG-2021-0063
52. Pervaiz S, Qureshi TA, Kashwani G, Kannan S. 3D printing of fiber-reinforced plastic composites using fused deposition modeling: A status review. Materials 2021; 14(16): 4520. doi: 10.3390/MA14164520
53. Wei X, Li D, Jiang W, et al. 3D printable graphene composite. Scientific Reports 2015; 5(1): 11181. doi: 10.1038/srep11181
54. Zhu D, Ren Y, Liao G, et al. Thermal and mechanical properties of polyamide 12/graphene nanoplatelets nanocomposites and parts fabricated by fused deposition modeling. Journal of Applied Polymer Science 2017; 134(39): 45332. doi: 10.1002/app.45332
55. Saadi MASR, Maguire A, Pottackal NT, et al. Direct ink writing: A 3D printing technology for diverse materials. Advanced Materials 2022; 34(28): 2108855. doi: 10.1002/ADMA.202108855
56. Singh M, Haverinen HM, Dhagat P, Jabbour GE. Inkjet printing—Process and its applications. Advanced Materials 2010; 22(6): 673–685. doi: 10.1002/adma.200901141
57. Lim S, Kang B, Kwak D, et al. Inkjet-printed reduced graphene oxide/poly(vinyl alcohol) composite electrodes for flexible transparent organic field-effect transistors. The Journal of Physical Chemistry C 2012; 116(13): 7520–7525. doi: 10.1021/jp203441e
58. García-Tuñon E, Barg S, Franco J, et al. Printing in three dimensions with graphene. Advanced Materials 2015; 27(10): 1688–1693. doi: 10.1002/adma.201405046
59. Uçak N, Çiçek A, Aslantas K. Machinability of 3D printed metallic materials fabricated by selective laser melting and electron beam melting: A review. Journal of Manufacturing Processes 2022; 80: 414–457. doi: 10.1016/J.JMAPRO.2022.06.023
60. Acord KA, Dupuy AD, Scipioni Bertoli U, et al. Morphology, microstructure, and phase states in selective laser sintered lithium ion battery cathodes. Journal of Materials Processing Technology 2021; 288: 116827. doi: 10.1016/J.JMATPROTEC.2020.116827
61. Zhou X, Nowicki M, Cui H, et al. 3D bioprinted graphene oxide-incorporated matrix for promoting chondrogenic differentiation of human bone marrow mesenchymal stem cells. Carbon 2017; 116: 615–624. doi: 10.1016/j.carbon.2017.02.049
62. Pagac M, Hajnys J, Ma QP, et al. A review of vat photopolymerization technology: Materials, applications, challenges, and future trends of 3D printing. Polymers 2021; 13(4): 598. doi: 10.3390/POLYM13040598
63. Costa BMDC, Griveau S, Bedioui F, et al. Stereolithography based 3D-printed microfluidic device with integrated electrochemical detection. Electrochimica Acta 2022; 407: 139888. doi: 10.1016/J.ELECTACTA.2022.139888
64. Li C, Du J, Gao Y, et al. Stereolithography of 3D sustainable metal electrodes towards high‐performance nickel iron battery. Advanced Functional Materials 2022; 32(40): 2205317. doi: 10.1002/ADFM.202205317
65. Gaikwad S, Tate JS, Theodoropoulou N, Koo JH. Electrical and mechanical properties of PA11 blended with nanographene platelets using industrial twin-screw extruder for selective laser sintering. Journal of Composite Materials 2013; 47(23): 2973–2986. doi: 10.1177/0021998312460560
66. Shuai C, Feng P, Gao C, et al. Graphene oxide reinforced poly(vinyl alcohol): Nanocomposite scaffolds for tissue engineering applications. RSC Advances 2015; 5: 25416–25423. doi: 10.1039/C4RA16702C
67. Zhai F, Feng Y, Li Z, et al. 4D-printed untethered self-propelling soft robot with tactile perception: Rolling, racing, and exploring. Matter 2021; 4(10): 3313–3326. doi: 10.1016/J.MATT.2021.08.014
68. Yu Y, Feng Y, Liu F, et al. Carbon dots-based ultrastretchable and conductive hydrogels for high-performance tactile sensors and self-powered electronic skin. Small 2023; 19(31): 2204365. doi: 10.1002/SMLL.202204365
69. Zhu Y, Tang T, Zhao S, et al. Recent advancements and applications in 3D printing of functional optics. Additive Manufacturing 2022; 52: 102682. doi: 10.1016/J.ADDMA.2022.102682
70. Jiang Y, Islam MN, He R, et al. Recent advances in 3D printed sensors: Materials, design, and manufacturing. Advanced Materials Technologies 2023; 8(2): 2200492. doi: 10.1002/ADMT.202200492
71. Tan HW, Choong YYC, Kuo CN, et al. 3D printed electronics: Processes, materials and future trends. Progress in Materials Science 2022; 127: 100945. doi: 10.1016/J.PMATSCI.2022.100945
72. Zhang F, Feng Y, Feng W. Three-dimensional interconnected networks for thermally conductive polymer composites: Design, preparation, properties, and mechanisms. Materials Science and Engineering: R: Reports 2020; 142: 100580. doi: 10.1016/J.MSER.2020.100580
73. Li Z, Wang L, Li Y, et al. Carbon-based functional nanomaterials: Preparation, properties and applications. Composites Science and Technology 2019; 179: 10–40. doi: 10.1016/J.COMPSCITECH.2019.04.028
74. Zhang D, Chi B, Li B, et al. Fabrication of highly conductive graphene flexible circuits by 3D printing. Synthetic Metals 2016; 217: 79–86. doi: 10.1016/j.synthmet.2016.03.014
75. Liu H, Zhang H, Han W, et al. 3D printed flexible strain sensors: From printing to devices and signals. Advanced Materials 2021; 33(8): 2004782. doi: 10.1002/ADMA.202004782
76. Schwierz F. Graphene transistors. Nature Nanotechnology 2010;5(7): 487–496. doi: 10.1038/nnano.2010.89
77. Xiang L, Wang Z, Liu Z, et al. Inkjet-printed flexible biosensor based on graphene field effect transistor. IEEE Sensors Journal 2016; 16(23): 8359–8364. doi: 10.1109/JSEN.2016.2608719
78. Huang L, Huang Y, Liang J, et al. Graphene-based conducting inks for direct inkjet printing of flexible conductive patterns and their applications in electric circuits and chemical sensors. Nano Research 2011; 4(7): 675–684. doi: 10.1007/s12274-011-0123-z
79. Willian MD, Michaela E, Mae CH, et al. A comprehensive review on the application of 3D printing in the aerospace industry. Key Engineering Materials 2022; 913: 27–34. doi: 10.4028/p-94a9zb
80. Mohanavel V, Ashraff Ali KS, Ranganathan K, et al. The roles and applications of additive manufacturing in the aerospace and automobile sector. Materials Today: Proceedings 2021; 47: 405–409. doi: 10.1016/J.MATPR.2021.04.596
81. Sugiyama K, Matsuzaki R, Ueda M, et al. 3D printing of composite sandwich structures using continuous carbon fiber and fiber tension. Composites Part A: Applied Science and Manufacturing 2018; 113: 114–121. doi: 10.1016/J.COMPOSITESA.2018.07.029
82. Sai Saran O, Prudhvidhar Reddy A, Chaturya L, Pavan Kumar M. 3D printing of composite materials: A short review. Materials Today: Proceedings 2022; 64: 615–619. doi: 10.1016/J.MATPR.2022.05.144
83. Wang X, Jin J, Song M. An investigation of the mechanism of graphene toughening epoxy. Carbon 2013; 65: 324–333. doi: 10.1016/j.carbon.2013.08.032
84. Verma M, Verma P, Dhawan SK, Choudhary V. Tailored graphene based polyurethane composites for efficient electrostatic dissipation and electromagnetic interference shielding applications. RSC Advances 2015; 5(118): 97349–97358. doi: 10.1039/C5RA17276D
85. Singh AP, Garg P, Alam F, et al. Phenolic resin-based composite sheets filled with mixtures of reduced graphene oxide, γ-Fe2O3 and carbon fibers for excellent electromagnetic interference shielding in the X-band. Carbon 2012; 50(10): 3868–3875. doi: 10.1016/j.carbon.2012.04.030
86. Fu K, Wang Y, Yan C, et al. Graphene oxide-based electrode inks for 3D-printed lithium-ion batteries. Advanced Materials 2016; 28(13): 2587–2594. doi: 10.1002/adma.201505391
87. Rocha VG, García-Tuñón E, Botas C, et al. Multimaterial 3D printing of graphene-based electrodes for electrochemical energy storage using thermoresponsive inks. ACS Applied Materials&Interfaces 2017; 9(42): 37136–37145. doi: 10.1021/acsami.7b10285
88. Shen K, Mei H, Li B, et al. 3D printing sulfur copolymer-graphene architectures for Li-S batteries. Advanced Energy Materials 2018; 8(4): 1701527. doi: 10.1002/aenm.201701527
89. Huang K, Yang J, Dong S, et al. Anisotropy of graphene scaffolds assembled by three-dimensional printing. Carbon 2018; 130: 1–10. doi: 10.1016/j.carbon.2017.12.120
90. Vernardou D, Vasilopoulos KC, Kenanakis G. 3D printed graphene-based electrodes with high electrochemical performance. Applied Physics A 2017; 123: 623. doi: 10.1007/s00339-017-1238-1
91. Fan D, Li Y, Wang X, et al. Progressive 3D printing technology and its application in medical materials. Frontiers in Pharmacology 2020; 11: 516624. doi: 10.3389/FPHAR.2020.00122/BIBTEX
92. Al-Dulimi Z, Wallis M, Tan DK, et al. 3D printing technology as innovative solutions for biomedical applications. Drug Discovery Today 2021; 26(2): 360–383. doi: 10.1016/J.DRUDIS.2020.11.013
93. Chadha U, Abrol A, Vora NP, et al. Performance evaluation of 3D printing technologies: A review, recent advances, current challenges, and future directions. Progress in Additive Manufacturing 2022; 7(5): 853–886. doi: 10.1007/S40964-021-00257-4
94. Mallakpour S, Tabesh F, Hussain CM. 3D and 4D printing: From innovation to evolution. Advances in Colloid and Interface Science 2021; 294: 102482. doi: 10.1016/J.CIS.2021.102482
95. Zhang L, Forgham H, Shen A, et al. Nanomaterial integrated 3D printing for biomedical applications. Journal of Materials Chemistry B 2022; 10(37): 7473–7490. doi: 10.1039/D2TB00931E
96. Kantaros A. 3D printing in regenerative medicine: Technologies and resources utilized. International Journal of Molecular Sciences 2022; 23(23): 14621. doi: 10.3390/IJMS232314621
97. Pavan Kalyan BG, Kumar L. 3D printing: Applications in tissue engineering, medical devices, and drug delivery. AAPS PharmSciTech 2022; 23(4): 1–20. doi: 10.1208/S12249-022-02242-8
98. Kalkal A, Kumar S, Kumar P, et al. Recent advances in 3D printing technologies for wearable (bio)sensors. Additive Manufacturing 2021; 46: 102088. doi: 10.1016/J.ADDMA.2021.102088
99. Bozkurt Y, Karayel E. 3D printing technology; methods, biomedical applications, future opportunities and trends. Journal of Materials Research and Technology 2021; 14: 1430–1450. doi: 10.1016/J.JMRT.2021.07.050
100. Wang W, Caetano G, Ambler WS, et al. Enhancing the hydrophilicity and cell attachment of 3D printed PCL/graphene scaffolds for bone tissue engineering. Materials (Basel) 2016; 9(12): 992. doi: 10.3390/ma9120992
101. Cheng Z, Landish B, Chi Z, et al. 3D printing hydrogel with graphene oxide is functional in cartilage protection by influencing the signal pathway of Rank/Rankl/OPG. Materials Science and Engineering: C 2018; 82: 244–252. doi: 10.1016/j.msec.2017.08.069
102. Chen Q, Mangadlao JD, Wallat J, et al. 3D printing biocompatible polyurethane/poly(lactic acid)/graphene oxide nanocomposites: Anisotropic properties. ACS Applied Materials & Interfaces 2017; 9(4): 4015–4023. doi: 10.1021/acsami.6b11793
103. Sayyar S, Bjorninen M, Haimi S, et al. Uv cross-linkable graphene/poly(trimethylene carbonate) composites for 3D printing of electrically conductive scaffolds. ACS Applied Materials & Interfaces 2016; 8(46): 31916–31925. doi: 10.1021/acsami.6b09962
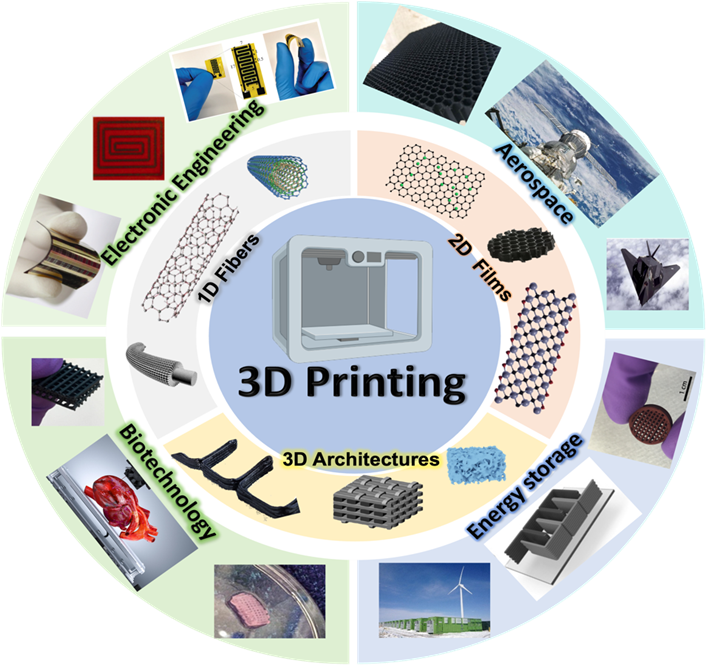
##submission.downloads##
已出版
文章引用
期
栏目
执照
版权声明
CC BY-NC 4.0