清洁能源的曙光:强化传热、辐射制冷与“鞭炮式”可控核聚变发电系统
DOI:
https://doi.org/10.18686/cncest.v1i1.46摘要
全球气候变暖已成为人类面临的重大环境威胁与发展挑战。可控核聚变是世界公认的清洁能源理想方案,但其所需的高能引发条件和剧烈能量释放,使现有技术都未能实现安全、稳定、长周期连续运行。为此,本团队受到中国传统鞭炮原理的启示,原创提出脉冲式聚变反应飞轮储能多反应堆接力运行,驱动汽轮机长周期持续稳定发电;在冷却介质管路中设置“洁能芯”以强化换热,并在冷却塔表面采用辐射制冷技术以提升冷却效率并降低能耗,从而提升系统安全性及整体能效。本文提出热端和冷端原创技术联用,努力为可控核聚变发电开辟一条崭新的途径。
参考
1. Guo L, Ou Z, Liu Y, et al. Technological innovations on direct carbon mitigation by ordered energy conversion and full resource utilization. Carbon Neutrality 2022; 1: 4. doi: 10.1007/s43979-022-00009-5
2. Wang N. Fusion Energy and Its Future (Chinese). Tsinghua University Press; 2001.
3. Lindl J. Development of the indirect-drive approach to inertial confinement fusion and the target physics basis for ignition and gain. Physics of Plasmas 1995; 2: 3933–4024. doi: 10.1063/1.871025
4. Fisch NJ. Plasma physics and controlled nuclear fusion. In: Libby SB, van Bibber KA (editors). Modern Physics and the Scientific Legacy of Edward Teller, Proceedings of the Edward Teller Centennial Symposium; 28 May 2008; California, USA. World Scientific; 2010.
5. Wesson J, Campbell DJ. Tokamaks. Oxford University Press; 2011.
6. Barabaschi P, Kamada Y, Shirai H, JT-60SA Integrated Project Team. Progress of the JT-60SA project. Nuclear Fusion 2019; 59(11): 112005. doi: 10.1088/1741-4326/ab03f6
7. Zhuang G, Li GQ, Li J, et al. Progress of the CFETR design. Nuclear Fusion 2019; 59(11): 112010. doi: 10.1088/1741-4326/ab0e27
8. Li J, Wan Y. Present state of Chinese magnetic fusion development and future plans. Journal of Fusion Energy 2019; 38: 113–124. doi: 10.1007/s10894-018-0165-2
9. Ye H. A brief analysis of EAST tokamak and related fusion research in China (Chinese). Science and Innovation 2021; 12: 92–93. doi: 10.15913/j.cnki.kjycx.2021.12.037
10. Pfalzner S. An Introduction to Inertial Confinement Fusion. CRC Press; 2006.
11. Zhao Y, Xiao G, Li F. Current status and development of inertial confinement fusion physics research based on modern gas pedals (Chinese). Physics 2016; 45(2): 98–107. doi: 10.7693/wl20160204
12. Basov NG, Ivanov YS, Krokhin ON. Neutron generation in spherical irradiation of a target by high-power laser radiation. Journal of Experimental and Theoretical Physics Letters 1972; 15(10): 417–419.
13. Pavlovskiĭ AI. Reminiscences of different years. Soviet Physics Uspekhi 1991; 34(5): 429. doi: 10.1070/PU1991v034n05ABEH002378
14. Gao S, Wu X, He Z, et al. Research progress of fabrication techniques for laser inertial confinement fusion target. High Power and Particle Beams 2020; 32: 032001. doi: 10.11884/HPLPB202032.200039
15. Huang H, Ma L. Current status of fusion energy development (Chinese). China Science and Technology Information 2023(4): 120–122, 125.
16. Yang W, Zhang E. Pulsed Microfluidic Fusion Impulse Flywheel Control Continuous Power Generation Technology (Chinese). CN Patent 116,110,617, 12 May 2023.
17. Yang W, Zhang E. Microfluidic Deuterium-Tritium Collision Nuclear Fusion Flywheel Control Continuous Power Generation Technology (Chinese). CN Patent 116,434,980, 14 July 2023.
18. Stacey WM. Fusion Plasma Physics, 2nd ed. Wiley-VCH; 2013.
19. Lyublinski IE, Vertkov AV. Experience and technical issues of liquid lithium application as plasma facing material in tokamaks. Fusion Engineering and Design 2010; 85(6): 924–929. doi: 10.1016/j.fusengdes.2010.08.036
20. Humrickhouse PW, Merrill BJ, Yoon SJ, Cadwallader LC. The impacts of liquid metal plasma-facing components on fusion reactor safety and tritium management. Fusion Science and Technology 2019; 75(8): 973–1001. doi: 10.1080/15361055.2019.1658464
21. Bergles AE. Advanced enhancement-third generation heat transfer technology or “the final frontier”. Transaction of the Institute of Chemistry Engineering 2001; 79 (Part A): 437–444.
22. Webb RL, Eckert ERG. Application of rough surfaces to heat exchanger design. International Journal of Heat and Mass Transfer 1972; 15(9): 1647–1658. doi: 10.1016/0017-9310(72)90095-6
23. Zimparov V. Extended performance evaluation criteria for enhanced heat transfer surfaces: Heat transfer through ducts with constant heat flux. International Journal of Heat and Mass Transfer 2001; 44(1): 169–180. doi: 10.1016/S0017-9310(00)00074-0
24. Wang S, Li ZX, Guo ZY. Novel concept and device of heat transfer augmentation. In: Proceedings of 11th International Conference of Heat transfer; 1 November 1998; Kyongju, Korea. Taylor Fancis; 1998. pp. 405–408.
25. Guo ZY, Li DY, Wang BX. A novel concept for convective heat transfer enhancement. International Journal of Heat and Mass Transfer 1998; 41(14): 2221–2225. doi: 10.1016/S0017-9310(97)00272-X
26. Guo Z. Physical mechanism of convective heat transfer and its control: Synergy between velocity and heat flow fields (Chinese). Science Bulletin 2000; 45(19): 2118–2122. doi: 10.3321/j.issn:0023-074X.2000.19.020
27. Guo Z, Wang S. Novel concept and approaches of heat transfer enhancement. In: Proceedings of Symposium on Energy Engineering in the 21st Century (SEE2000) Volume I–IV. Begell House; 2002. pp. 118–126. doi: 10.1615/SEE2000.90
28. Tao W, He Y, Huang P. Numerical validation of field synergy theory in an alternating flow slit-type heat rector (Chinese). Journal of Engineering Thermophysics 2003; 24(4): 649–651.
29. He Y, Lei Y, Tian L, et al. Exploration of the three-field synergy of high-efficiency low-resistance enhanced heat transfer technology (Chinese). Journal of Engineering Thermophysics 2009; 30(11): 1904–1906.
30. Xia X, Zhao L, Xu H, Yang S. Comprehensive performance evaluation factors for enhanced heat transfer based on field synergy theory (Chinese). Thermodynamic Engineering 2011; 26(2): 197–201.
31. Zhang Z. Research on Heat Transfer Enhancement Mechanism and Performance of Assembled Rotors (Chinese) [PhD thesis]. Beijing University of Chemical Technology; 2014.
32. Li Y, Ding Y, Yang W. Numerical analysis of the working principle of cleaning rotors and its industrial application (Chinese). Petrochemical Equipment 2009; 38(6):73–76.
33. Yang W, Li F, Chen S, Yan H. Industrial experimental study of combined pipe-end rotor enhanced heat transfer device (Chinese). Thermodynamic Engineering 2008; 23(4): 378–381.
34. Li FX, Ding YM, Guan CF, et al. Laboratory investigation and commercial test for rotors-assembled strand applied in smooth tube. Experimental Thermal and Fluid Science 2008; 33(1): 1–9. doi: 10.1016/j.expthermflusci.2008.04.005
35. Cui Y, Luo X, Zhang F, et al. Progress of passive daytime radiative cooling technologies towards commercial applications. Particuology 2022; 67: 57–67. doi: 10.1016/J.PARTIC.2021.10.004
36. Li T, Zhai Y, He S, et al. A radiative cooling structural material. Science 2019; 364(6442): 760–763. doi: 10.1126/science.aau9101
37. Yin X, Yang R, Tan G, Fan S. Terrestrial radiative cooling: Using the cold universe as a renewable and sustainable energy source. Science 2020; 370(6518): 786–791. doi: 10.1126/science.abb0971
38. Wang H, Xue C, Guo X, et al. Superhydrophobic porous film for daytime radiative cooling. Applied Materials Today 2021; 24: 101100. doi:10.1016/J.APMT.2021.101100
39. Zhong H, Li Y, Zhang P, et al. Hierarchically hollow microfibers as a scalable and effective thermal insulating cooler for buildings. ACS Nano 2021; 15(6): 10076–10083. doi: 10.1021/ACSNANO.1C01814
40. Liang J, Wu J, Guo J, et al. Radiative cooling for passive thermal management towards sustainable carbon neutrality. National Science Review 2023: 10(1): nwac208. doi: 10.1093/NSR/NWAC208
41. Poredoš P, Wang R. Sustainable cooling with water generation. Science 2023; 380(6644): 458–459. doi: 10.1126/science.add1795
42. Cai C, Chen W, Wei Z, et al. Bioinspired “aerogel grating” with metasurfaces for durable daytime radiative cooling for year-round energy savings. Nano Energy 2023; 114: 108625. doi: 10.1016/J.NANOEN.2023.108625
43. Li J, Fu Y, Zhou J, et al. Ultrathin, soft, radiative cooling interfaces for advanced thermal management in skin electronics. Science Advances 2023; 9(14): eadg1837. doi: 10.1126/sciadv.adg1837
44. Fixen DJ. The temperature of the cosmic microwave background. The Astrophysical Journal 2009; 707(2): 916–920. doi: 10.1088/0004-637X/707/2/916
45. Hossain MM, Gu M. Radiative cooling: Principles, progress, and potentials. Advanced Science 2016; 3(7): 1500360. doi: 10.1002/advs.201500360
46. Zhao D, Aili A; Zhai Y, et al. Radiative sky cooling: Fundamental principles, materials, and applications. Applied Physics Reviews 2019; 6(2): 021306. doi: 10.1063/1.5087281
47. Farooq AS, Zhang P, Gao Y, Gulfam R. Emerging radiative materials and prospective applications of radiative sky cooling—A review. Renewable and Sustainable Energy Reviews 2021; 144: 110910. doi: 10.1016/J.RSER.2021.110910
48. Raman AP, Anoma MA, Zhu L, et al. Passive radiative cooling below ambient air temperature under direct sunlight. Nature 2014; 515(7528): 540–544. doi: 10.1038/nature13883
49. Zhai Y, Ma Y, David SN, et al. Scalable-manufactured randomized glass-polymer hybrid metamaterial for daytime radiative cooling. Science 2017; 355(6329): 1062–1066. doi: 10.1126/science.aai7899
50. Tian Y, Liu X, Wang Z, et al. Subambient daytime cooling enabled by hierarchically architected all-inorganic metapaper with enhanced thermal dissipation. Nano Energy 2022; 96: 107085. doi: 10.1016/J.NANOEN.2022.107085
51. Chen Y, Dang B, Fu J, et al. Cellulose-based hybrid structural material for radiative cooling. Nano Letters 2021; 21(1): 397–404. doi: 10.1021/ACS.NANOLETT.0C03738
52. Son S, Jeon S, Chae D, et al. Colored emitters with silica-embedded perovskite nanocrystals for efficient daytime radiative cooling. Nano Energy 2020; 79: 105461. doi: 10.1016/j.nanoen.2020.105461
53. Li X, Peoples J, Yao P, Ruan X. Ultrawhite BaSO4 paints and films for remarkable daytime subambient radiative cooling. ACS Applied Materials & Interfaces 2021; 13(18): 21733–21739. doi: 10.1021/ACSAMI.1C02368
54. Gao H, Zhang F, Tang K, et al. Green cleaning of 3D-printed polymeric products by micro-/nano-bubbles. Nanomaterials 2023; 13(11): 1804. doi: 10.3390/NANO13111804
55. Zhou L, Li Z. Exploring the standard system of micro bubble technology. Water Purification Technology 2021; 40: 75–87.
56. Zhang L, Chen H, Li Z. Long lifetime of nanobubbles due to high inner density. Science in China Series G: Physics Mechanics & Astronomy 2008; 51(2): 219–224. doi: 10.1007/s11433-008-0026-5
57. Yu Z, Li J, Zhang X. A new hypothesis for cavitation nucleation in gas saturated solutions: Clustering of gas molecules lowers significantly the surface tension. Chinese Journal of Chemical Engineering 2022; 50: 347–351. doi: 10.1016/J.CJCHE.2022.06.009
58. Li P, Wang J, Liao Z. Microbubbles for effective cleaning of metal surfaces without chemical agents. Langmuir 2022; 38(2): 769–776. doi: 10.1021/ACS.LANGMUIR.1C02769
59. Zhang R, Gao Y, Chen L, et al. Nanobubble boundary layer thickness quantified by solvent relaxation NMR. Journal of Colloid and Interface Science 2022; 609: 637–644. doi: 10.1016/J.JCIS.2021.11.072
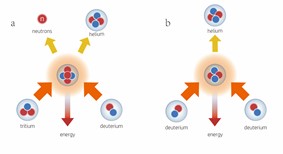
##submission.downloads##
已出版
文章引用
期
栏目
执照
版权声明
CC BY-NC 4.0